西安城区黑碳气溶胶的污染特征及来源解析
Characteristics and source analysis of black carbon aerosol in Xi'an Urban Area
-
摘要: 为了了解西安城区的黑碳污染特征及潜在来源,利用AE-31黑碳仪于2015年3月-2016年2月在西安观测的黑碳(BC)质量浓度,得到西安市区BC质量浓度的变化特征,并基于黑碳仪模型以及浓度权重轨迹分析(CWT)对BC排放来源和潜在源区进行了分析.结果表明,观测期间西安市区BC质量浓度的变化范围为0.5-17.4 μg·m3,平均值为(4.1±2.9)μg·m3.BC平均浓度季节变化呈现出冬季(5.2±4.1)μg·m-3 > 秋季(4.7±3.7)μg·m-3 > 春季(3.2±2.4)μg·m-3 > 夏季(2.8±1.9)μg·m-3的递减趋势.BC浓度与温度呈负相关,这主要受冬季燃煤增加及不利气象条件的影响;其与能见度、降水和风速均呈负相关.各季节BC浓度日变化趋势基本一致,主要受边界层、交通排放及人类活动的影响,峰谷值分别出现在06:00-09:00和14:00-17:00时间段.由黑碳仪模型可知,BCff和BCbb在春、夏、秋、冬4个季节BC总浓度中占比分别71.9%和28.1%、75.5%和24.5%、76.2%和23.8%、54.8%和45.2%;由波长吸收指数(AAE)的分析可知,春、夏、秋、冬4个季节的AAE平均值分别为1.22±0.11、1.11±0.13、1.16±0.14和1.45±0.12.结合BCff,BCbb在4个季节中的贡献和AAE结果可知,西安市大气中的BC污染主要源自于化石燃料的燃烧,冬季生物质燃烧对BC的贡献增加.由CWT的结果分析可知,影响西安市BC高值区主要集中在西安南部和西南部.Abstract: To investigate the characteristics and potential sources of black carbon (BC) pollution in Xi'an, the mass concentrations of BC were measured from March 2015 to February 2016 in a urban site using AE-31 Aethalometer were used to obtain the characteristics of the variation of BC mass concentrations in Xi'an City, and the BC emission sources and potential source areas were analyzed based on the aethalometer model and concentration weighted trajectory analysis (CWT). The results showed that BC mass concentrations varied from 0.5 to 17.4 μg·m-3 during the sampling period, with a mean value of (4.1±2.9) μg·m-3. The seasonal averaged BC concentrations showed a decreased trend of winter (5.2±4.1)μg·m-3 > autumn (4.7±3.7)μg·m-3 > spring (3.2±2.4) μg·m-3 > summer (2.8±1.9) μg·m-3. BC was negatively correlated with temperature, which is mainly affected by the increase in coal burning in winter and adverse weather conditions, and it was negatively correlated with visibility, precipitation and wind speed. In general, diurnal variations of BC are consistent among different seasons, suggesting BC concentrations were mainly influenced by the boundary layer, traffic emissions and human activities. The peak and valley values appeared in the time period of 06:00-09:00 and 14:00-17:00 in all seasons, respectively. According to the "aethalometer model" method, BCff account for 71.9%, 75.5%, 76.2% and 54.8%, while BCbb account for 28.1%, 24.5%, 23.8% and 45.2% of the total BC concentrations in spring, summer, autumn and winter, respectively. The analysis of Absorption Angstrom Exponent (AAE) showed that the averaged AAEs of spring, summer, autumn and winter are 1.22±0.11, 1.11±0.13, 1.16±0.14 and 1.45±0.12, respectively. Combined the contributions of BCff, BCbb in four seasons and the results of AAEs, we conclude that the BC in Xi'an mainly come from fossil fuels combustion. Moreover, the contribution of biomass burning to BC increased in winter. The concentration-weighted trajectory (CWT) analysis result showed that the south and southwest directions of Xi'an are the potential source regions for high concentration of BC.
-
[1] WANG J, LIU D, GE X, et al. Characterization of black carbon-containing fine particles in Beijing during wintertime[J]. Atmospheric Chemistry and Physics, 2019, 19(1):447-458. [2] 许黎, 王亚强, 陈振林,等. 黑碳气溶胶研究进展Ⅰ:排放、清除和浓度[J]. 地球科学进展, 2006(4):352-360. XU L, WANG Y Q, CHEN Z L, et al. Progress of black carbon aerosol research I:Emission, removal and concentration[J]. Advances in Earth Science, 2006 (4):352-360(in Chinese).
[3] HUANG R J, ZHANG Y, BOZZETTI C, et al. High secondary aerosol contribution to particulate pollution during haze events in China[J]. Nature, 2014, 514(7521):218-222. [4] SEINFELD J H. Air pollution:A half century of progress[J]. American Institute of Chemical Engineers, 2004,50(6):1096-1108. [5] WANG Y, ZHANG R Y & SARAVANAN R. Asian pollution climatically modulates midlatitude cyclones following hierarchical modeling and observational analysis[J]. Nature Communications, 2014, 5:1098. [6] CAO J J. Pollution status and control strategies of PM2.5 in China[J]. Journal of Earth Environment, 2012, 3:1030-1036. [7] BOND T C. Bounding the role of black carbon in the climate system:A scientific assessment[J]. Journal of Geophysical Research Atmospheres, 2013, 118(11):5380-5552. [8] DING A J, HUANG X, NIE W, et al. Enhanced haze pollution by black carbon in megacities in China[J]. Geophysical Research Letters, 2016, 43(6):2873-2879. [9] LEE A K Y, RIVELLINI L H, CHEN C L, et al. Influences of primary emission and secondary coating formation on the particle diversity and mixing state of black carbon particles[J]. Environmental Science & Technology, 2019, 53(16):9429-9438. [10] LIU C, XU X, YIN Y, et al. Black carbon aggregates:A database for optical properties[J]. Journal of Quantitative Spectroscopy and Radiative Transfer, 2019, 222/223:170-179. [11] XIAO S, WANG Q Y, CAO J J, et al. Long-term trends in visibility and impacts of aerosol composition on visibility impairment in Baoji, China[J]. Atmospheric Research, 2014, 149:88-95. [12] HUANG L,GONG S L,SHARMA S,et al. A trajectory analysis of atmospheric transport of black carbon aerosols to Canadian high Arctic in winter and spring (1990-2005)[J].Atmospheric Chemistry & Physics,2010,10(11):1716-1726. [13] GRAF H F, SHIRSAT S V, OPPENHEIMER C, et al. Continental Scale Antarctic deposition of sulphur and black carbon from anthropogenic and volcanic sources[J]. Atmospheric Chemistry & Physics, 2010, 10(5):2457-2465. [14] ZHUANG B L, WANG T J, LIU J, et al. Continuous measurement of black carbon aerosol in urban Nanjing of Yangtze River Delta, China[J]. Atmospheric Environment, 2014, 89:415-424. [15] 王洪强,贺千山,陈勇航,等.2008-2012年上海黑碳浓度变化特征分析[J].环境科学,2014,35(4):1215-1222. WANG H Q, HE Q S, CHEN Y H, et al. Analysis of characteristics of black carbon concentration in Shanghai from 2008 to 2012[J]. Environmental Science, 2014, 35(4):1215-1222(in Chinese).
[16] 谢锋,林煜棋,宋文怀,等.南京北郊黑碳气溶胶分布特征及来源[J].环境科学, 2020,41(10):4392-4401. XIE F,LIN Y Q, SONG W H. et al. Distribution characteristics and source of black carbon aerosols in the northern suburbs of Nanjing[J].Environmental Science, 2020,41(10):4392-4401(in Chinese).
[17] GAO R X, NIU S J, ZHANG H, et al. A comparative study on black carbon aerosol observations in regions of Beijing and Lhasa in 2006[C]//Conference on Remote Sensing and Modelling of Ecosystems for Sustainability Ⅳ, 2007. [18] XIE C, XU W, WANG J, et al. Vertical characterization of aerosol optical properties and brown carbon in winter in urban Beijing, China[J]. Atmospheric Chemistry and Physics, 2019, 19(1):165-179. [19] WU D, WU C, LIAO B, et al. Black carbon over the South China Sea and in various continental locations in South China[J]. Atmospheric Chemistry & Physics, 2013, 13(24):12257-12270. [20] CHEN X, ZHANG Z, ENGLING G, et al. Characterization of fine particulate black carbon in Guangzhou, a megacity of south China[J]. Atmospheric Chemistry and Physics, 2014, 5(3):361-370. [21] 王璐,袁亮,张小玲,等.成都地区黑碳气溶胶变化特征及其来源解析[J].环境科学,2020,41(4):1561-1572. WANG L, YUAN L, ZHANG X L, et al. Characteristics and source apportionment of black carbon in Chengdu[J]. Environmental Science, 2020,41(4):1561-1572(in Chinese).
[22] 肖思晗, 于兴娜, 朱彬, 等. 南京北郊黑碳气溶胶的来源解析[J]. 环境科学, 2018, 39(1):9-17. XIAO S H, YU X N, ZHU B, et al. Source apportionment of black carbon aerosol in the North Suburb of Nanjing[J]. Environmental Science, 2018, 39(1):9-17(in Chinese).
[23] 程丁, 吴晟, 吴兑, 等. 深圳市城区和郊区黑碳气溶胶对比研究[J]. 中国环境科学, 2018, 38(5):1653-1662. CHEN D, WU C, WU D, et al. Comparative study on the characteristics of black carbon aerosol in urban and suburban areas of Shenzhen[J]. China Environmental Science, 2018, 38(5):1653-1662(in Chinese).
[24] 赵锦慧,何超,李小莉,等.武汉市黑碳气溶胶质量浓度空间分布的主导因素分析[J].生态环境学报,2018,27(6):1089-1098. ZHAO J H, HE C, LI X L, et al. Leading factor analysis of horizontal and vertical distribution of black carbon aerosol in Wuhan[J]. Ecology and Environmental Sciences, 2018, 27(6):1089-1098(in Chinese).
[25] 张昕, 李忠勤, 明镜, 等. 乌鲁木齐河源区黑碳气溶胶浓度特征及其来源分析[J]. 环境科学学报, 2019, 39(1):212-221. ZHANG X, LI Z Q, MING J, et al. Characteristics of black carbon aerosol and source apportionment at the headwater of Urumqi River[J]. Acta Scientiae Circumstantiae, 2019, 39(1):212-221(in Chinese).
[26] 杨晓旻,施双双,张晨,等.南京市黑碳气溶胶时间演变特征及其主要影响因素[J].环境科学,2020,41(2):620-629. YANG X M, SHI S S, ZHANG C, et al. Time evolution characteristics and main influencing factors of black carbon aerosol in Nanjing[J]. Environmental Science, 2020, 41(2):620-629(in Chinese).
[27] 齐孟姚,王丽涛,张城瑜,等. 邯郸市黑碳气溶胶浓度变化及影响因素分析[J].环境科学学报,2018,38(5):1751-1758. QI M Y, WANG L T, ZHANG C Y, et al. Variation of black carbon aerosol concentration and its influencing factors in Handan City, Hebei Province[J]. Acta Scientiae Circumstantiae, 2018, 38(5):1751-1758(in Chinese).
[28] 牛晓婧. 西安市明城墙区域慢行交通系统研究[D].西安:长安大学,2015. ZHU X J. Study on the slow traffic system in Ming City area of Xi'an[D]. Xi'an:Chang'an University, 2015(in Chinese). [29] 朱崇抒, 曹军骥, 沈振兴, 等. 西安黑碳气溶胶的污染特征及其成因分析[J]. 中国粉体技术, 2009, 15(2):66-71. ZHU C S, CAO J J, SHEN Z X, et al. Variability of black carbon aerosol and analysis of influence factors over Xi'an atmosphere[J]. China Powder Science and Technology, 2009, 15(2):66-71(in Chinese).
[30] 杜川利, 余兴, 李星敏, 等. 西安泾河夏季黑碳气溶胶及其吸收特性的观测研究[J]. 中国环境科学, 2013, 33(4):613-622. DU C X, YU X, LI X M, et al. Observed summer variation and absorption characteristics of black carbon at Xi'an suburban[J]. China Environmental Science, 2013, 33(4):613-622(in Chinese).
[31] 刘立忠, 王宇翔, 么远, 等. 西安市黑碳气溶胶浓度特征及与气象因素和常规污染物相关性[J]. 中国环境监测, 2016, 32(5):45-50. LIU L Z, WANG Y X, YAO Y, et al. Concentration characteristics and the correlations of black carbon aerosols with meteorological factors and conventional pollutants in Xi'an[J]. Environmental Monitoring in China, 2016, 32(5):45-50(in Chinese).
[32] CAO J J, ZHU C S, CHOW J C, et al. Black carbon relationships with emissions and meteorology in Xi'an, China[J]. Atmospheric Research, 2009, 94(2):194-202. [33] ZHANG Q, SHEN Z X, NING Z, et al. Characteristics and source apportionment of winter black carbon aerosols in two Chinese megacities of Xi'an and Hong Kong[J]. Environmental Science & Pollution Research, 2018, 25(33):33783-33793. [34] WEINGARTNER E, SAATHOFF H, SCHNAITER M, et al. Absorption of light by soot particles:Determination of the absorption coefficient by means of aethalometers[J]. Journal of Aerosol Science, 2003, 34(10):1445-1463. [35] VIRKKULA A, MÄKELÄ T, HILLAMO R, et al. A simple procedure for correcting loading effects of Aethalometer Data[J]. Journal of the Air & Waste Management Association, 2007,57(10):12-14. [36] WU D, WU C, LIAO B, et al. Seasonal variation of black carbon over the South China Sea and in various continental locations in South China[J]. Atmospheric Chemistry and Physics Discussions, 2013,13(7):17375-17405. [37] WU C, WU D, YU J Z. Quantifying black carbon light absorption enhancement by a novel statistical approach[J]. Atmospheric Chemistry & Physics, 2018, 18(1):289-309. [38] SANDRADEWI J, PRÉVÔT A S, SZIDAT S, et al. Using aerosol light absorption measurements for the quantitative determination of wood burning and traffic emission contributions to particulate matter[J]. Environmental Science & Technology, 2008,42(9):3316-3323. [39] KIRCHSTETTER T W,NOVAKOV T,HOBBS P V. Evidence that the spectral dependence of light absorption by aerosols is affected by organic carbon[J].Journal of Geophysical Research,2004,109(D21):D21208. [40] 李莉,蔡鋆琳,周敏.2013年12月中国中东部地区严重灰霾期间上海市颗粒物的输送途径及潜在源区贡献分析[J].环境科学,2015,36(7):2327-2336. LI L,CAI J L,ZHOU M. Potential source contribution analysis of the particulate matters in Shanghai during the heavy haze episode in eastern and middle China in December,2013[J].Environmental Science,2015,36(7):2327-2336(in Chinese).
[41] KOMPALLI S K,BABU S S,MOORTHY K K,et al.Aerosol black carbon characteristics over Central India:Temporal variation and its dependence on mixed layer height[J]. Atmospheric Research, 2014,147:27-37. [42] WANG Y Q, ZHANG X Y, DRAXLER R R. TrajStat:GIS-based software that uses various trajectory statistical analysis methods to identify potential sources from long-term air pollution measurement data[J]. Environmental Modelling & Software, 2009, 24(8):938-939. [43] 王绪鑫,马雁军,向旬,等.鞍山黑碳气溶胶观测[J].环境化学,2010,29(6):1091-1095. WANG X X, MA Y J, XIANG X, et al. Observational of black carbon aerosol in Anshan[J]. Environmental Science, 2010, 29(6):1091-1095(in Chinese).
[44] 黄观,刘伟,刘志红,等.黑碳气溶胶研究概况[J].灾害学,2015,30(2):205-214. HUANG G, LIU W, LIU Z H, et al. A research overview of Black Carbon Aerosols[J]. Journal of Catastrophology, 2015, 30(2):205-214.
[45] KIRCHSTETTER T W. Evidence that the spectral dependence of light absorption by aerosols is affected by organic carbon[J]. Journal of Geophysical Research Atmospheres, 2004, 109(D21):1-12. [46] RUSSELL P B,BERGSTROM R W,SHINOZUKA Y,et al.Absorption angstrom exponent in AERONET and related data as an indicator of aerosol composition[J]. Atmospheric Chemistry and Physics,2010, 10(3):1155-1169. [47] 程丁,吴晟,吴兑,等.广州市城区干湿季黑碳气溶胶污染特征及来源分析[J].环境科学学报,2018,38(6):2223-2232. CHENG D, WU C, WU D, et al. Characteristics of black carbon aerosols in urban Guangzhou:Influencing factors in dry and rainy seasons[J].Acta Scientiae Circumstantiae,2018,38(6):2223-2232(in Chinese).
[48] 陈慧忠,吴兑,廖碧婷,等.东莞与帽峰山黑碳气溶胶浓度变化特征的对比[J].中国环境科学,2013,33(4):605-612. CHEN H Z, WU D, LIAO B J, et al. Compare of black carbon concentration variation between dongguan and maofengshan[J]. China Environmental Science, 2013,33(4):605-612(in Chinese).
[49] ZHUANG B L, WANG T J, LIU J, et al. Absorption coefficient of urban aerosol in Nanjing, west Yangtze River Delta, China[J]. Atmospheric Chemistry and Physics, 2015, 15(23):13633-13646. [50] SUN J, SHEN Z, CAO J, et al. Particulate matters emitted from maize straw burning for winter heating in rural areas in Guanzhong Plain, China:Current emission and future reduction[J]. Atmos[pheric Research, 2017, 184:66-76. -
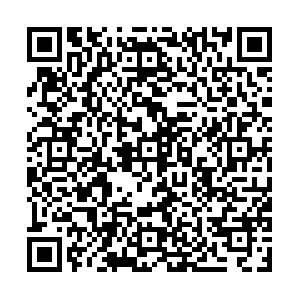
计量
- 文章访问数: 2549
- HTML全文浏览数: 2549
- PDF下载数: 109
- 施引文献: 0