透明胞外聚合颗粒物及其膜污染机理的研究进展
A review of transparent exopolymer particles and their membrane fouling mechanisms
-
摘要: 透明胞外聚合颗粒物(transparent exopolymer particles,TEP)是一类主要成分为酸性多糖的高黏性有机微凝胶,普遍存在于海水、淡水和废水中,影响碳元素、微生物和颗粒物等在水环境中的迁移循环.TEP是膜分离系统中一种重要的有机污染物,在过滤过程中附着在膜表面或黏附在膜孔内壁,显著增加膜阻力.研究显示,水环境中的藻类和细菌的种类和生长阶段等因素影响TEP的形成和含量.TEP与膜表面生物膜的形成和早期发育密切相关,是膜生物污染形成的主要成因.颗粒态TEP易在微滤、超滤和反渗透膜表面形成滤饼层,而胶体态TEP和TEP前体易阻塞膜孔或通过超微滤膜孔在反渗透膜表面形成凝胶层.电解质能促进胶体态TEP凝聚形成颗粒态TEP减轻超滤膜污染,同时也能被超滤膜截留去除.絮凝、沉淀、过滤等工艺组合可有效去除TEP,更好地控制膜污染.Abstract: Transparent exopolymer particles (TEP) are highly viscous organic microgels and consist predominantly of acidic polysaccharide. TEP are ubiquitous in most source waters, such as sea, surface water, ground water and wastewater, etc. They affect the migration and circulation of carbon, microorganisms and particles in various water environments. TEP have been identified as important organic foulants in membrane systems. They can attach on the membrane surface or on the inner walls of membrane pores during filtration, significantly increasing the membrane resistance. In order to understand membrane fouling induced by TEP in membrane filtration process, the definition, biotic and abiotic formation and determination methods of TEP were introduced, and the membrane fouling mechanisms of TEP were discussed in this paper. The research evidence showed that the species and growth stages of algae and bacteria influenced the formation of TEP which led to serious membrane fouling. It was identified that TEP correlated strongly with the formation and early development of biofilm, which could potentially lead to biofouling. The processes of flocculation, sedimentation and filtration could improve the TEP removal efficiency and substantially reduce TEP-associated fouling. Finally, the further research areas of TEP in membrane separation process were proposed.
-
Key words:
- transparent exopolymer particles /
- membrane fouling /
- gel layer /
- biofilm /
- biofouling
-
[1] LIN H, ZHANG M, WANG F, et al. A critical review of extracellular polymeric substances (EPSs) in membrane bioreactors:Characteristics, roles in membrane fouling and control strategies[J]. Journal of Membrane Science, 2014, 460(12):110-125. [2] WANG Z, WU Z, YIN X, et al. Membrane fouling in submerged membrane bioreactor (MBR) under subscritical flux operation:membrane foulant and gel layer characterization[J]. Journal of Membrane Science, 2008, 325(2):238-244. [3] GAO D, FU Y, REN N. Tracing biofouling to the structure of the microbial community and its metabolic products:A study of the three-stage MBR process[J]. Water Research, 2013, 47(17):6680-6690. [4] LIANG S, ZHAO Y, LIU C, et al. Effect of solution chemistry on the fouling potential of dissolved organic matter in membrane bioreactor system[J]. Journal of Membrane Science, 2008, 310(1/2):502-511. [5] TANG S, WANG Z, WU Z, et al. Role of dissolved organic matter (DOM) in membrane fouling of membrane bioreactores for municipal wastewater treatement[J]. Journal of Hazardous Materials, 2010, 178:377-384. [6] LISHMAN L, AQEEL H, BASUVARAJ M, et al. Biofouling of an aerated membrane reactor:Four distinct microbial communities[J]. Environmental Engineering Science, 2020, 37(1):3-12. [7] BAR-ZEEV E, BERMAN-FRANK I, LIBERMAN B, et al. Transparent exopolymer particles:Potential agents for organic fouling and biofilm formation in desalination and water treatment plants[J]. Desalination and Water Treatment, 2009, 3:136-142. [8] BAR-ZEEV E, BERMAN-FRANK I, STAMBLER N, et al. Transparent exopolymer particles (TEP) link phytoplankton and bacterial production in the Gulf of Aqaba[J]. Aquatic Microbial Ecology, 2009, 56(2/3):217-225. [9] AMY G. Fundamental understanding of organic matter fouling of membranes[J]. Desalination, 2008, 231:44-51. [10] LI S, WINTERS H, JEONG S, et al. Marine bacterial transparent exopolymer particles (TEP) and TEP precursors:Characterization and RO fouling potential[J]. Desalination, 2016, 379(3):68-74. [11] PASSOW U, SWEET J, FRANCIS S, et al. Incorporation of oil into diatom aggregates[J]. Marine Ecology Progress Series, 2019, 612(1):65-86. [12] PASSOW U. Transparent exopolymer particles (TEP) in aquatic environments[J]. Progress in Oceanography, 2002, 55(3/4):287-333. [13] PASSOW U, SHIPE R F, MURRAY A, et al. The origin of transparent exopolymer particles (TEP) and their role in the sedimentation of particulate matter[J]. Continental Shelf Research, 2001, 21(4):327-346. [14] PASSOW U. Production of transparent exopolymer particles (TEP) by phyto-and bacterioplankton[J]. Marine Ecology Progress Series, 2002, 236(11):1-12. [15] JENNINGS M K, PASSOW U, WOZNIAK A S, et al. Distribution of transparent exopolymer particles (TEP) across an organic carbon gradient in the western North Atlantic Ocean[J]. Marine Chemistry, 2017, 190(3):1-12. [16] CHIN W C, ORELLANA M V, VERDUGO P. Spontaneous assembly of marine dissoved organic matter into polymer gels[J]. Nature, 1998, 391(6667):568-572. [17] BAR-ZEEV E, BERMAN T, RAHAV E, et al. Teansparent expolymer particles (TEP) dynamics in the eastern Mediterranean Sea[J]. Marine Ecology Progress Series, 2011, 431(6):107-118. [18] HONG Y, SMITH W O, WHITE A M. Studies on transparent exopolymer particles (TEP) produced in the Ross Sea (Antarctica) and by Phaeocystis antarctica (Prymnesiophyceae)[J]. Journal of Phycology, 1997, 33(3):368-376. [19] MARI X, PASSOW U, MIGON C, et al. Transparent exopolymer particles:Effects on carbon cycling in the ocean[J]. Progress in Oceanography, 2017, 151(2):13-37. [20] AZETSU-SCOTT K, PASSOW U. Ascending marine particles:Significance of transparent exopolymer particles (TEP) in the upper ocean[J]. Limnology and Oceanography, 2004, 49(3):741-748. [21] ROBINSON T B, STOLLE C, WURL O. Depth is relative:The importance of depth for transparent exopolymer particles in the near-surface environment[J]. Ocean Science, 2019, 15(6):1653-1666. [22] BERMAN T, HOLENBERG M A. Don't fall foul of biofilm through high TEP levels[J]. Filtration & Separation, 2005, 42(4):30-32. [23] BERMAN T. Biofouling:TEP-a major challenge for water filtration[J]. Filtration & Separation, 2010, 47(2):20-22. [24] TORRE T D L, LESJEAN B, DREWS A, et al. Monitoring of transparent exopolymer particles (TEP) in a membrane bioreactor (MBR) and correlation with other fouling indicators[J]. Water Science and Technology, 2008, 58(10):1903-1909. [25] VILLACORTE L O, SCHURER R, KENNEDY M D, et al. Removal and deposition of transparent exopolymer particles in a seawater UF-RO system[J]. IDA Journal of Desalination and Water Reuse, 2010, 2(1):45-55. [26] KENNEDY M D, TOBAR F P M, AMY G, et al. Transparent exopolymer particle (TEP) fouling of ultrafiltration membrane systems[J]. Desalination and Water Treatment, 2009, 6:169-176. [27] BERMAN T, VINER-MOZZINI Y. Abundance and characteristics of polysaccharide and proteinaceous particles in Lake Kinneret[J]. Aquatic Microbial Ecology, 2001, 24(3):255-264. [28] PASSOW U, ALLDREDGE A L. Aggregation of a diatom bloom in a mesocosm:The role of transparent exopolymer particles (TEP)[J]. Deep-Sea Research Part Ⅱ:Tropical Studies in Oceanography, 1995, 42(1):99-109. [29] 马丽丽, 陈敏, 郭劳动, 等. 北白令海透明胞外聚合颗粒物的含量与来源[J]. 海洋学报(中文版), 2012, 34(5):81-90. MA L, CHEN M, GUO L, et al. Distribution and source of tranapartent exopolymer particles in the northern Bering Sea[J]. Acta Oceanologica Sinica, 2012, 34(5):81-90(in Chinese). [30] THORNTON D C O. Formation of transparent exopolymeric particles (TEP) from macroalgal detritus[J]. Marine Ecology Progress Series, 2004, 282(17):1-12. [31] NEVEL S V, HENNEBEL T, BEUF K D, et al. Transparent exopolymer particle removal in different drinking water production centers[J]. Water Research, 2012, 46(11):3603-3611. [32] BAR-ZEEV E, BERMAN-FRANK I, GIRSHEVITZ O, et al. Revised paradigm of aquatic biofilm formation facilitated by microgel transparent exopolymer particles[J]. Proceedings of the National Academy of Sciences of the United States of America, 2012, 109(23):9119-9124. [33] BERMAN T, PASSOW U. Transparent exopolymer particles (TEP):An overlooked factor in the process of biofilm formation in aquatic environments[J]. Nature Precedings, 2007, DOI:10.1038/npre.2007.1182.1 [34] SANTSCHI P H, BALNOIS E, WILKINSON K J, et al. Fibrillar polysaccharides in marine macromolecular organic matter as imaged by atomic force microscopy and transmission electron microscopy[J]. Limnology and Oceanography, 1998, 43(5):896-908. [35] LI S, WINTERS H, VILLACORTE L O, et al. Compositional similarities and differences between transparent exopolymer particles (TEPs) from two marine bacteria and two marine algae:Significience to surface biofouling[J]. Marine Chemistry, 2015, 174(7):131-140. [36] PASSOW U, ALLDREDGE A L. A dye-binding assay for the spectrophotometric measurement of transparent exopolymer particles (TEP)[J]. Limnology and Oceanography, 1995, 40(7):1326-1335. [37] ALLDREDGE A L, PASSOW U, LOGAN B E. The abundance and significance of a class of large, transparent organic particles in the ocean[J]. Deep Sea Research Part I:Oceanographic Resarch Papers, 1993, 40(6):1131-1140. [38] XU C, CHIN W C, LIN P, et al. Comparison of microgels, extracellular polymeric substances (EPS) and transparent exopolymeric particles (TEP) determined in seawater with and without oil[J]. Marine Chemistry, 2019, 215(8):103667. [39] 刘丽贞, 黄琪, 秦伯强, 等. 透明胞外聚合颗粒物在水处理中的应用研究进展[J]. 水处理技术, 2015, 41(12):15-19. LIU L, HUANG Q, QIN B, et al. Advances in the application of transparent exopolymer particle (TEP) in water treatment[J]. Technology of Water Treatment, 2015, 41(12):15-19(in Chinese).
[40] BERMAN T. Transparent exopolymer particles as critical agents in aquatic biofilm formation:implications for desalination and water treatment[J]. Desalination and Water Treatment, 2013, 51:1014-1020. [41] STODEREGGER K E, HERNDL G J. Production of exopolymer particles by marine bacterioplankton under contrasting turbulence conditions[J]. Marine Ecology Progress Series, 1999, 189(14):9-16. [42] RADIĆ T, KRAUS R, FUKS D, et al. Transparent exopolymeric particles' distribution in the northern Adriatic and their relation to microphytoplankton biomass and composition[J]. Science of the Total Environment, 2005, 353(1):151-161. [43] PRAIRIE J C, MONTGOMERY Q W, PROCTOR K W, et al. Effects of phytoplankton growth phase on settling properties of marine aggregates[J]. Journal of Marine Science and Engineering, 2019, 7(8):265. [44] 郭康丽, 陈洁, 王小冬, 等. 两种海洋硅藻透明胞外聚合颗粒物的产生及其生态学意义[J]. 海洋环境科学, 2019, 38(5):649-655. GUO K L, CHEN J, WANG X D, et al. Production of transparent exopolymer particles from two marine diatoms and its ecological significance[J]. Chinese Journal of Marine Environmental Science, 2019, 38(5):649-655(in Chinese).
[45] FUKAO T, KIMOTO K, KOTANI Y. Produciton of transparent exopolymer particles by four diatom species[J]. Fisheries Science, 2010, 76(5):755-760. [46] 孙翠慈, 王友绍, 吴梅林, 等. 夏季珠江口透明胞外聚合颗粒物分布特征[J]. 热带海洋学报, 2010, 29(5):81-87. SUN C C, WANG Y S, WU M L, et al. Distribution of transparent exopolymer particles in the pearl River Estuary in summer[J]. Journal of Tropical Oceanography, 2010, 29(5):81-87(in Chinese).
[47] GUERRINI F, MAZZOTTI A, BONI L, et al. Bacterial-algal interactions in polysaccharide production[J]. Aquatic Microbial Ecology, 1998, 15(3):247-253. [48] BERMAN-FRANK I, ROSENBERG G, LEVITAN O, et al. Coupling between autocatalytic cell death and transparent exopolymeric particle production in the marine cyanobacterium Trichodesmium[J]. Environmental Microbiology, 2007, 9(6):1415-1422. [49] ENGEL A, PASSOW U. Carbon and nitrogen content of transparent exopolymer particles (TEP) in relation to their alcian blue adsorption[J]. Marine Ecology Progress Series, 2001, 219(11):1-10. [50] PASSOW U. Formation of transparent exoplymer particles TEP, from dissoloved precursor material[J]. Marine Ecology Progress Series, 2000, 192(1):1-11. [51] CHANDRASEKARAN R, RADHA A. Molecular modeling of xanthan:Galactomannan interactions[J]. Carbohydrate Polymer, 1997, 32(3):201-208. [52] MENG S, WINTERS H, LIU Y. Ultrafiltration behaviors of alginate blocks at various calcium concentration[J]. Water Research, 2015, 83(16):248-257. [53] WANG R, LIANG D, LIU X, et al. Effect of magnesium ion on polysaccharide fouling[J]. Chemical Engineering Journal, 2020, 379(1):122351. [54] VILLACORTE L O, KENNEDY M D, AMY G L, et al. The fate of transparent exopolymer particles (TEP) in integrated membrane systems:Removal through pre-treatment processes and deposition on reverse osmosis membranes[J]. Water Research, 2009, 43(20):5039-5053. [55] VILLACORTE L O, SCHURER R, KENNEDY M D, et al. The fate of transparent exopolymer particles (TEP) in seawater UF-RO system:A pilot plant study in Zeeland, The Netherlands[J]. Desalination and Water Treatment, 2010, 13:109-119. [56] LI X, SKILLMAN L, LI D, et al. Comparison of alcian blue and total carbohydrate assays for quantitation of transparent exopolymer particles (TEP) in biofouling studies[J]. Water Research, 2018, 133(6):60-68. [57] MENG S, RZECHOWICZ M, WINTERS H, et al. Transparent exopolymer particles (TEP) and their potential effect on membrane biofouling[J]. Applied Microbiology and Biotechnology, 2013, 97(13):5705-5710. [58] MENG S, FAN W, LI X, et al. Intermolecular interactions of polysaccharides in membrane fouling during microfiltration[J]. Water Research, 2018, 143(16):38-46. [59] LI S, LEE S T, SINHA S, et al. Transparent exopolymer particles (TEP) removal efficiency by a combination of coagulation and ultrafiltration to minimize SWRO membrane fouling[J]. Water Research, 2016, 102(15):485-493. [60] KOMLENIC R. Rethinking the causes of membrane biofouling[J]. Filtration & Separation, 2010, 47(5):26-28. [61] MENG S, LIU Y. New insights into transparent exopolymer particles (TEP) formation from precursor materials at various Na+/Ca2+ ratios[J]. Scientific Reports, 2016, 6(1):19747. [62] SIM L N, SUWARNO S R, LEE D Y S, et al. Online monitoring of transparent exopolymer particles (TEP) by a novel membrane-based spectrophotometric method[J]. Chemosphere, 2019, 220(7):107-115. [63] BERMAN T, PARPAROVA R. Visualization of transparent exopolymer particles (TEP) in various source waters[J]. Desalination and Water Treatment, 2010, 21:382-289. [64] WETZ M S, ROBBINS M C, PAERL H W. Transparent exopolymer particles (TEP) in a river-dominated estuary:Spatial-temporal distributions and an assessment of controls upon TEP formation[J]. Estuaries and Coasts, 2009, 32(3):447-455. [65] SUN C C, WANG Y S, LI Q P, et al. Distribution characteristics of transparent exopolymer particles in the Pearl River estuary, China[J]. Journal of Geophysical Research:Biogeosciences, 2012,G00N17,doi:10.1029/2012JG001951. [66] ZAMANILLO M, ORTEGA-RETUERTA E, NUNES S, et al. Distribution of transparent exopolymer particles (TEP) in distinct regions of the Southern Ocean[J]. Science of the Total Environment, 2019, 691(46):736-748. [67] GUO S, SUN X. Concentrations and distribution of transparent exopolymer particles in a eutrophic coastal sea:A case study of the Changjiang (Yangtze River) estuary[J]. Marine & Freshwater Research, 2019, 70(10):1389-1401. [68] 舒逸, 张桂成, 孙军. 东海PN断面透明胞外聚合颗粒物分布特征及来源研究[J]. 海洋学报, 2018, 40(8):110-119. SHU Y, ZHANG G, SUN J. The distribution and origin of transparent exopolymer particles at the PN section in the East China Sea[J]. Acta Oceanologica Sinica, 2018, 40(8):110-119(in Chinese).
[69] RIEBESELL U, REIGSTAD M, WASSMANN P, et al. On the trophic fate of Phaeocystis pouchetii (Hariot):VI. significience of Phaeocystis-derived mucus for vertical flux[J]. Nertherlands Journal of Sea Research, 1995, 33(2):193-203. [70] PRIETO L, COWEN J P. Transparent exopolymer particles in a deep-sea hydrothermal system:Guaymas Basin, Gulf of California[J]. Marine Biology, 2007, 150(6):1093-1101. [71] ENGEL A. Distribution of transparent exopolymer particles (TEP) in the northeast Atlantic Ocean and their potential significance for aggregation processes[J]. Deep-Sea Research Part I, 2003, 51(1):83-92. [72] GROSSART H P, SIMON M, LOGAN B E. Formation of macroscopic organic aggregates (lake snow) in a large lake:The significance of transparent exopolymer particles, plankton, and zooplankton[J]. Limnology and Oceanography, 1997, 42(8):1651-1659. [73] DISCART V, BILAD M R, NEVEL S V, et al. Role of transparent exopolymer particles on membrane fouling in a full-scale ultrafiltration plant:Feed parameter analysis and membrane autopsy[J]. Bioresource Technology, 2014, 173(23):67-74. [74] MARI X, BURD A. Seasonal size spectra of transparent exopolymeric particles (TEP) in a coastal sea and comparison with those predicted using coagulation theory[J]. Marine Ecology Progress Series, 1998, 163(2):63-76. [75] VICENTE I D, ORTEGA-RETUERTA E, MAZUECOS I P, et al. Variation in transparent exopolymer particles in relation to biological and chemical factors in two contrasting lake districts[J]. Aquatic Sciences, 2010, 72(4):443-453. [76] HUANG Q, LIU L, QIN B, et al. Abundance, characteristics, and size spectra of transparent exopolymer particles and coomassie stainable particles during spring in a large shallow lake, Taihu, China[J]. Journal of Great Lakes Research, 2016, 42(2):455-463. [77] ARNOUS M B, COURCOL N, CARRIAS J F. The significance of transparent exopolymeric particles in the vertical distribution of bacteria and heterotrophic nanoflagellates in Lake Pavin[J]. Aquatic Sciences, 2010, 72(2):245-253. [78] ORTEGA-RETUERTA E, DUARTE C M, RECHE I. Significance of bacterial activity for the distribution and dynamics of transparent exopolymer particles in the Mediterranean Sea[J]. Microbial Ecology, 2010, 59(4):808-818. [79] ORTEGA-RETUERTA E, MARRAS C, MU OZ-FERN NDEZ A, et al. Seasonal dynamics of transparent exopolymer particles (TEP) and their drivers in the coastal NW Mediterranean Sea[J]. Science of the Total Environment, 2018, 631/632(16):180-190. [80] 彭安国, 黄奕普. 九龙江河口区TEP及其与铀、钍、钋同位素相关性的研究[J]. 厦门大学学报(自然科学版), 2007, 46(Z1):38-42. PENG A G, HUANG Y P. Study on TEP and its relationships with uranium, thorium, polonium isotopes in Jiulong Estuary[J]. Journal of Xiamen University(Natural Science), 2007, 46(Z1):38-42(in Chinese). [81] PARINOS C, GOGOU A, KRASAKOPOULOU E, et al. Transparent exopolymer particles (TEP) in the NE Aegean Sea frontal area:Seasonal dynamics under the influence of Black Sea water[J]. Continental Shelf Research, 2017, 149(18):112-123. [82] NAGARAJ V, SKILLMAN L, LI D, et al. Review-bacteria and their extracellular polymeric substances causing biofouling on seawater reverse osmosis desalination membranes[J]. Journal of Environmental Management, 2018, 223(19):586-599. [83] HABIMANA O, SEMIAO A J C, CASEY E. The role of cell-surface interations in bacterial initial adhesion and consequent biofilm formation on nanofiltration/reverse osmosis membrane[J]. Journal of Membrane Science, 2014, 454(6):82-96. [84] LEE H, PARK C, KIM H, et al. Role of transparent exopolymer particles (TEP) in initial bacterial deposition and biofilm formation on reverse osmosis (RO) membrane[J]. Journal of Membrane Science, 2015, 494(22):25-31. [85] VERDUGO P, ALLDREDGE A L, AZAM F, et al. The oceanic gel phase:A bridge in the DOM-POM continuum[J]. Marine Chemistry, 2004, 92:67-85. [86] BAR-ZEEV E, PASSOW U, CASTRILL N S R V, et al. Transparent exopolymer particles:From aquatic environments and engineered systems to membrane biofouling[J]. Environmental Science & Technology, 2015, 49(2):691-707. [87] FLEMMING H C, WINGENDER J. The bioflim matrix[J]. Nature Reviews Microbiology, 2010, 8(9):623-633. [88] YIN X, LI X, HUA Z, et al. The growth process of the cake layer and membrane fouling alleviation mechanism in a MBR assisted with the self-generated electric field[J]. Water Research, 2020, 171(4):115452. [89] BERMAN T, MIZRAHI R, DOSORETZ C G. Transparent exopolymer particles (TEP):A critical factor in aquatic biofilm initiation and fouling on filtration membranes[J]. Desalination, 2011, 276:184-190. [90] ZHANG Z, CHEN M, LI J, et al. Significance of transparent exopolymer particles derived from aquatic algae in membrane fouling[J]. Arabian Journal of Chemistry, 2020, 13(3):4577-4585. [91] LI S, HEIJMAN S G J, VERBERK J Q J C, et al. Fouling control mechanisms of demineralized water backwash:Reduction of charge screenning and calcium bridging effects[J]. Water Research, 2011, 45(19):6289-6300. [92] VILLACORTE L O, KENNEDY M D, AMY G L, et al. Measuring transparent exopolymer particles (TEP) as indicator of the (bio)fouling potential of RO feed water[J]. Desalination and Water Treatment, 2009, 5:207-212. [93] LIN J C T, WU C Y, CHU Y L, et al. Effects of high turbidity seawater on removal of boron and transparent exopolymer particles by chemical oxo-precipitation[J]. Journal of the Taiwan Institute of Chemical Engineers, 2019, 94(1):109-118. [94] SHAO S, FU W, LI X, et al. Membrane fouling by the aggregations formed from oppositely charged organic foulants[J]. Water Research, 2019, 159(12):95-101. -
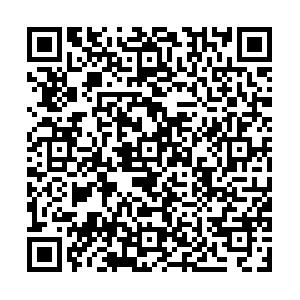
计量
- 文章访问数: 3208
- HTML全文浏览数: 3208
- PDF下载数: 55
- 施引文献: 0