室内环境中传统与新型溴代阻燃剂的采样方法研究进展
Sampling methods for legacy and novel brominated flame retardants in indoor environment
-
摘要: 溴代阻燃剂(brominated flame retardants,BFRs)是一类性质稳定、效果出色的阻燃剂,作为工业添加剂被广泛应用于生活用品、电子电器以及织物的生产过程中,在全球正被大量使用.由于这些产品大多在室内使用,是BFRs人体暴露的主要来源.近年来,传统与新型BFRs在室内环境中常被同时检出,且BFRs在室内环境中的浓度普遍比室外更高,因此,BFRs在室内环境中的污染特征与暴露风险受到越来越多的关注.本文对近年来全球范围内室内环境中BFRs的研究作了汇总与梳理,对比采样方法的差异,评价方法的适用环境.针对室内空气,被动采样与小流量主动采样都有普遍的应用,但由于被动采样耗时长,在目前的研究中更多用于浓度较低的室内环境;而小流量主动采样,虽然灵活性低,但采样效率高,在目前的研究中更多用于高浓度的室内环境.室内灰尘的采集方式大体上包括吸尘器和刷子采样,其中真空吸尘器采样效率高,虽然被普遍应用,但对灰尘扰动性强,有可能低估一些挥发性较强的低溴BFRs的浓度水平;因而,刷子也常作为室内灰尘的采样手段,虽然效率较低,但更多用于大量积尘的台面,能够降低对于细颗粒物的扰动.目前我国已成为室内环境中BFRs的主要研究地区之一,但针对新型BFRs的调查研究还不够全面,应加强对于室内空气和灰尘的多介质监测研究.因此,本文展望室内环境中BFRs采样手段应在保证满足多种类目标化合物的同步监测的同时,提高对于BFRs化合物的采集选择性,并鼓励更多空气与灰尘样品的配套分析研究,以期对我国室内环境中BFRs的调查研究手段提供参考.Abstract: Brominated Flame Retardants (BFRs) are a series of stable and outstanding flame retardants and have been used in large quantities worldwide in manufacturing processes of human daily uses, electronics and textiles. These products are mostly used in indoor environment thus become main human exposure sources. So far, legacy and novel BFRs have been frequently detected in indoor environment with levels higher than those in outdoor environment. As a result, contamination characteristics and human exposure risks of BFRs in indoor environment are gaining increasing concerns. This study summaries studies in recent years to compare their sampling methods and evaluate the suitable conditions. As for indoor air, passive sampling and low-volume active sampling are both widely used. Passive sampling takes more time and has been adopted more frequently in the indoor environment of comparatively low BFR concentrations. Low-volume active sampling is less flexible but with good efficiency, and it is deployed more often in the indoor environment of comparatively high BFR concentrations in the literature. Sampling methods for indoor dust usually take vacuum cleaners and brushes. Despite of frequent application and high efficiency, vacuum cleaners may strongly remobilize precipitated dust, thus possibly lead to underestimated levels of low-brominated compounds, which are of higher volatility. Although with relatively low efficiency, brushes are often used to sample elevated surfaces with amounted dust without strongly remobilizing fine particles. For now, China has become one of the largest regions for studies on contamination characteristics of BFRs in indoor environment, but more researches on novel BFRs in indoor environment are needed to promote monitoring of multiple matrices for indoor air and dust. Therefore, this study proposes outlooks that methods of indoor sampling should focus on improving selectivity on BFR sampling while pursuing simultaneously monitoring multi-classes compounds and more researches are also encouraged for paired indoor air and dust samples, in expect to share fundamental ideas for study methodologies on BFRs in indoor environment in China.
-
Key words:
- BFRs /
- indoor air /
- indoor dust /
- sampling method /
- contamination characteristics
-
[1] COVACI A, HARRAD S, ABDALLAH M A, et al. Novel brominated flame retardants:A review of their analysis, environmental fate and behaviour[J]. Environ Int, 2011, 37(2):532-556. [2] DARNERUD P O, ERIKSEN G S, JOHANNESSON T, et al. Polybrominated diphenyl ethers:Occurrence, dietary exposure, and toxicology[J]. Environ Health Persp, 2001, 109:49-68. [3] DE WIT C A. An overview of brominated flame retardants in the environment[J]. Chemosphere, 2002, 46(5):583-624. [4] DARNERUD P. Toxic effects of brominated flame retardants in man and in wildlife[J]. Environment International, 2003, 29(6):841-853. [5] MCDONALD T A. A perspective on the potential health risks of PBDEs[J]. Chemosphere, 2002, 46(5):745-755. [6] 田亚静.六溴环十二烷修正案对我国的影响及对策建议[J]. 生态经济, 2017, 33(12):180-183. TIAN Y J. The impact and the countermeasures of the HBCD amendment in China[J]. Ecological Economy, 2017, 33(12):180-183(in Chinese).
[7] LAW R J, COVACI A, HARRAD S, et al. Levels and trends of PBDEs and HBCDs in the global environment:Status at the end of 2012[J]. Environ Int, 2014, 65:147-158. [8] LI W L, LIU L Y, SONG W W, et al. Five-year trends of selected halogenated flame retardants in the atmosphere of Northeast China[J]. Sci Total Environ, 2016, 539:286-293. [9] LI Q L, YANG K, LI K C, et al. New halogenated flame retardants in the atmosphere of nine urban areas in China:Pollution characteristics, source analysis and variation trends[J]. Environmental Pollution, 2017, 224:679-688. [10] HAN W L, FENG J L, GU Z P, et al. Polybrominated diphenyl ethers in the atmosphere of Taizhou, a major e-waste dismantling area in China[J]. B Environ Contam Tox, 2009, 83(6):783-788. [11] DING N, WANG T, CHEN S J, et al. Brominated flame retardants (BFRs) in indoor and outdoor air in a community in Guangzhou, a megacity of southern China[J]. Environ Pollut, 2016, 212:457-463. [12] MOLLER A, XIE Z, STURM R, et al. Polybrominated diphenyl ethers (PBDEs) and alternative brominated flame retardants in air and seawater of the European Arctic[J]. Environ Pollut, 2011, 159(6):1577-1583. [13] UENO D, ISOBE T, RAMU K, et al. Spatial distribution of hexabromocyclododecanes (HBCDs), polybrominated diphenyl ethers (PBDEs) and organochlorines in bivalves from Japanese coastal waters[J]. Chemosphere, 2010, 78(10):1213-1219. [14] GAO S T, WANG J Z, YU Z Q, et al. Hexabromocyclododecanes in surface soils from e-waste recycling areas and industrial areas in South China:Concentrations, diastereoisomer- and enantiomer-specific profiles, and inventory[J]. Environmental Science & Technology, 2011, 45(6):2093-2099. [15] LI W L, MA W L, ZHANG Z F, et al. Occurrence and source effect of novel brominated flame retardants (NBFRs) in soils from five asian countries and their relationship with PBDEs[J]. Environ Sci Technol, 2017, 51(19):11126-11135. [16] WU M H, HAN T, XU G, et al. Occurrence of hexabromocyclododecane in soil and road dust from mixed-land-use areas of Shanghai, China, and its implications for human exposure[J]. Science of the Total Environment, 2016, 559:282-290. [17] MENG X Z, DUAN Y P, YANG C, et al. Occurrence, sources, and inventory of hexabromocyclododecanes (HBCDs) in soils from Chongming Island, the Yangtze River Delta (YRD)[J]. Chemosphere, 2011, 82(5):725-731. [18] GU S Y, EKPEGHERE K I, KIM H Y, et al. Brominated flame retardants in marine environment focused on aquaculture area:Occurrence, source and bioaccumulation[J]. Sci Total Environ, 2017, 601/602:1182-1191. [19] XIAO H, SHEN L, SU Y, et al. Atmospheric concentrations of halogenated flame retardants at two remote locations:The canadian high arctic and the tibetan plateau[J]. Environ Pollut, 2012, 161:154-161. [20] DE WIT C A, HERZKE D, VORKAMP K. Brominated flame retardants in the Arctic environment-trends and new candidates[J]. Sci Total Environ, 2010, 408(15):2885-2918. [21] DE WIT C A, ALAEE M, MUIR D C. Levels and trends of brominated flame retardants in the Arctic[J]. Chemosphere, 2006, 64(2):209-233. [22] DE WIT C A, HERZKE D, VORKAMP K. Brominated flame retardants in the Arctic environment-trends and new candidates[J]. Science of the Total Environment, 2010, 408(15):2885-2918. [23] SUN J, CHEN Q, HAN Y, et al. Emissions of selected brominated flame retardants from consumer materials:The effects of content, temperature, and timescale[J]. Environ Sci Pollut Res Int, 2018, 25(24):24201-24209. [24] VOJTA S, MELYMUK L, KLANOVA J. Changes in flame retardant and legacy contaminant concentrations in indoor air during building construction, furnishing, and use[J]. Environ Sci Technol, 2017, 51(20):11891-11899. [25] HARRAD S, ABDALLAH M A. Brominated flame retardants in dust from UK cars-within-vehicle spatial variability, evidence for degradation and exposure implications[J]. Chemosphere, 2011, 82(9):1240-1245. [26] KALACHOVA K, HRADKOVA P, LANKOVA D, et al. Occurrence of brominated flame retardants in household and car dust from the Czech Republic[J]. Sci Total Environ, 2012, 441:182-193. [27] CUNHA S C, KALACHOVA K, PULKRABOVA J, et al. Polybrominated diphenyl ethers (PBDEs) contents in house and car dust of Portugal by pressurized liquid extraction (PLE) and gas chromatography-mass spectrometry (GC-MS)[J]. Chemosphere, 2010, 78(10):1263-1271. [28] BESIS A, SAMARA C. Polybrominated diphenyl ethers (PBDEs) in the indoor and outdoor environments——a review on occurrence and human exposure[J]. Environ Pollut, 2012, 169:217-229. [29] WONG F, DE WIT C A, NEWTON S R. Concentrations and variability of organophosphate esters, halogenated flame retardants, and polybrominated diphenyl ethers in indoor and outdoor air in Stockholm, Sweden[J]. Environ Pollut, 2018, 240:514-522. [30] CHEN S J, DING N, ZHU Z C, et al. Sources of halogenated brominated retardants in house dust in an industrial city in southern China and associated human exposure[J]. Environ Res, 2014, 135:190-195. [31] CAO Z, ZHAO L, MENG X, et al. Amplification effect of haze on human exposure to halogenated flame retardants in atmospheric particulate matter and the corresponding mechanism[J]. J Hazard Mater, 2018, 359:491-499. [32] STUBBINGS W A, NGUYEN L V, ROMANAK K, et al. Flame retardants and plasticizers in a Canadian waste electrical and electronic equipment (WEEE) dismantling facility[J]. Sci Total Environ, 2019, 675:594-603. [33] LI J, DONG Z, WANG Y, et al. Human exposure to brominated flame retardants through dust in different indoor environments:Identifying the sources of concentration differences in hair from men and women[J]. Chemosphere, 2018, 205:71-79. [34] GRAVEL S, LAVOUÉ J, BAKHIYI B, et al. Halogenated flame retardants and organophosphate esters in the air of electronic waste recycling facilities:Evidence of high concentrations and multiple exposures[J]. Environment International, 2019, 128:244-253. [35] OKEME J O, YANG C, ABDOLLAHI A, et al. Passive air sampling of flame retardants and plasticizers in Canadian homes using PDMS, XAD-coated PDMS and PUF samplers[J]. Environ Pollut, 2018, 239:109-117. [36] STUBBINGS W A, SCHREDER E D, THOMAS M B, et al. Exposure to brominated and organophosphate ester flame retardants in U.S. childcare environments:Effect of removal of flame-retarded nap mats on indoor levels[J]. Environ Pollut, 2018, 238:1056-1068. [37] HE C, WANG X, THAI P, et al. Organophosphate and brominated flame retardants in Australian indoor environments:Levels, sources, and preliminary assessment of human exposure[J]. Environ Pollut, 2018, 235:670-679. [38] TAO S, CAO J, WANG W T, et al. A passive sampler with improved performance for collecting gaseous and particulate phase polycyclic aromatic hydrocarbons in air[J]. Environmental Science & Technology, 2009, 43(11):4124-4129. [39] ZHANG X M, DIAMOND M L, ROBSON M, et al. sources, emissions, and fate of polybrominated diphenyl ethers and polychlorinated biphenyls indoors in Toronto, Canada[J]. Environmental Science & Technology, 2011, 45(8):3268-3274. [40] BATTERMAN S A, CHERNYAK S, JIA C R, et al. Concentrations and emissions of polybrominated diphenyl ethers from US houses and garages[J]. Environmental Science & Technology, 2009, 43(8):2693-2700. [41] YADAV I C, DEVI N L, LI J, et al. Occurrence and source apportionment of halogenated flame retardants in the indoor air of Nepalese cities:Implication on human health[J]. Atmospheric Environment, 2017, 161:122-131. [42] KIM S K, PARK J E. Comparison of two different passive air samplers (PUF-PAS versus SIP-PAS) to determine time-integrated average air concentration of volatile hydrophobic organic pollutants[J]. Ocean Sci J, 2014, 49(2):137-150. [43] WILCOCKSON J B, GOBAS F A P. Thin-film solid-phase extraction to measure fugacities of organic chemicals with low volatility in biological samples[J]. Environmental Science & Technology, 2001, 35(7):1425-1431. [44] PERSSON J, WANG T, HAGBERG J. Temporal trends of decabromodiphenyl ether and emerging brominated flame retardants in dust, air and window surfaces of newly built low-energy preschools[J]. Indoor Air, 2019, 29(2):263-275. [45] TUDURI L, MILLET M, BRIAND O, et al. Passive air sampling of semi-volatile organic compounds[J]. TrAC Trends in Analytical Chemistry, 2012, 31:38-49. [46] HAZRATI S, HARRAD S. Calibration of polyurethane foam (PUF) disk passive air samplers for quantitative measurement of polychlorinated biphenyls (PCBs) and polybrominated diphenyl ethers (PBDEs):Factors influencing sampling rates[J]. Chemosphere, 2007, 67(3):448-455. [47] VENIER M, HITES R A. Flame retardants in the atmosphere near the Great Lakes[J]. Environmental Science & Technology, 2008, 42(13):4745-4751. [48] YANG Z, LIN T, CHENG H R, et al. Concentration and seasonal variation of halogenated flame retardants at a CAWNET background site in Central China[J]. Aerosol and Air Quality Research, 2018, 18(12):3068-3080. [49] YANG M, QI H, JIA H L, et al. Polybrominated diphenyl ethers in air across China:Levels, compositions, and gas-particle partitioning[J]. Environ Sci Technol, 2013, 47(15):8978-8984. [50] TOMS L M, BARTKOW M E, SYMONS R, et al. Assessment of polybrominated diphenyl ethers (PBDEs) in samples collected from indoor environments in South East Queensland, Australia[J]. Chemosphere, 2009, 76(2):173-178. [51] BJORKLUND J, TOLLBACK P, OSTMAN C. Large volume injection GC-MS in electron capture negative ion mode utilizing isotopic dilution for the determination of polybrominated diphenyl ethers in air[J]. J Sep Sci, 2003, 26(12/13):1104-1110. [52] MELYMUK L, BOHLIN-NIZZETTO P, PROKES R, et al. Sampling artifacts in active air sampling of semivolatile organic contaminants:Comparing theoretical and measured artifacts and evaluating implications for monitoring networks[J]. Environ Pollut, 2016, 217:97-106. [53] NGUYEN L V, DIAMOND M L, VENIER M, et al. Exposure of Canadian electronic waste dismantlers to flame retardants[J]. Environ Int, 2019, 129:95-104. [54] GUO J, STUBBINGS W A, ROMANAK K, et al. Alternative flame retardant, 2,4,6-tris(2,4,6-tribromophenoxy)-1,3,5-triazine, in an e-waste recycling facility and house dust in North America[J]. Environ Sci Technol, 2018, 52(6):3599-3607. [55] ZHANG M, SHI J, MENG Y, et al. Occupational exposure characteristics and health risk of PBDEs at different domestic e-waste recycling workshops in China[J]. Ecotox Environ Safe, 2019, 174:532-539. [56] DIE Q, NIE Z, HUANG Q, et al. Concentrations and occupational exposure assessment of polybrominated diphenyl ethers in modern Chinese e-waste dismantling workshops[J]. Chemosphere, 2019, 214:379-388. [57] VENIER M, AUDY O, VOJTA S, et al. Brominated flame retardants in the indoor environment-Comparative study of indoor contamination from three countries[J]. Environ Int, 2016, 94:150-160. [58] SUN J, WANG Q, ZHUANG S, et al. Occurrence of polybrominated diphenyl ethers in indoor air and dust in Hangzhou, China:Level, role of electric appliances, and human exposure[J]. Environ Pollut, 2016, 218:942-949. [59] HAN W, FAN T, XU B, et al. Passive sampling of polybrominated diphenyl ethers in indoor and outdoor air in Shanghai, China:seasonal variations, sources, and inhalation exposure[J]. Environ Sci Pollut Res Int, 2016, 23(6):5771-5781. [60] SJÖDIN A, CARLSSON H A, THURESSON K, et al. Flame retardants in indoor air at an electronics recycling plant and at other work environments[J]. Environmental Science & Technology, 2001, 35(3):448-454. [61] ZHU Y S, YANG W D, LI X W, et al. Airborne particle-bound brominated flame retardants:Levels, size distribution and indoor-outdoor exchange[J]. Environ Pollut, 2018, 233:1104-1112. [62] XU F, TANG W, ZHANG W, et al. Levels, distributions and correlations of polybrominated diphenyl ethers in air and dust of household and workplace in Shanghai, China:Implication for daily human exposure[J]. Environ Sci Pollut Res Int, 2016, 23(4):3229-3238. [63] HARNER T, SHOEIB M. Measurements of octanol-air partition coefficients (KOA) for polybrominated diphenyl ethers (pbdes):Predicting partitioning in the environment[J]. Journal of Chemical & Engineering Data, 2002, 47(2):228-232. [64] GOSS K U, ARP H P H, BRONNER G, et al. Partition behavior of hexachlorocyclohexane isomers[J]. Journal of Chemical & Engineering Data, 2008, 53(3):750-754. [65] QI H, LI W L, LIU L Y, et al. Brominated flame retardants in the urban atmosphere of Northeast China:Concentrations, temperature dependence and gas-particle partitioning[J]. Sci Total Environ, 2014, 491/492:60-66. [66] WESCHLER C J, NAZAROFF W W. Semivolatile organic compounds in indoor environments[J]. Atmospheric Environment, 2008, 42(40):9018-9040. [67] VORKAMP K, THOMSEN M, FREDERIKSEN M, et al. Polybrominated diphenyl ethers (PBDEs) in the indoor environment and associations with prenatal exposure[J]. Environ Int, 2011, 37(1):1-10. [68] STAPLETON H M, ALLEN J G, KELLY S M, et al. Alternate and new brominated flame retardants detected in US house dust[J]. Environmental Science & Technology, 2008, 42(18):6910-6916. [69] YAO Y, ZHAO Y, SUN H, et al. Per- and Polyfluoroalkyl Substances (PFASs) in indoor air and dust from homes and various microenvironments in China:Implications for human exposure[J]. Environ Sci Technol, 2018, 52(5):3156-3166. [70] STAAF T, OSTMAN C. Indoor air sampling of organophosphate triesters using solid phase extraction (SPE) adsorbents[J]. J Environ Monit, 2005, 7(4):344-348. [71] JAHNKE A, HUBER S, TEMME C, et al. Development and application of a simplified sampling method for volatile polyfluorinated alkyl substances in indoor and environmental air[J]. J Chromatogr A, 2007, 1164(1/2):1-9. [72] LAZAROV B, SWINNEN R, SPRUYT M, et al. Air sampling of flame retardants based on the use of mixed-bed sorption tubes-a validation study[J]. Environ Sci Pollut Res Int, 2015, 22(22):18221-18229. [73] LYCHE J L, ROSSELAND C, BERGE G, et al. Human health risk associated with brominated flame-retardants (BFRs)[J]. Environ Int, 2015, 74:170-180. [74] NIU D, QIU Y, DU X, et al. Novel brominated flame retardants in house dust from Shanghai, China:Levels, temporal variation, and human exposure[J]. Environmental Sciences Europe, 2019, 31(1):1-9. [75] JIN M T, LI L J, ZHENG Y X, et al. Polybrominated diphenyl ethers (PBDEs) in dust in typical indoor public places in Hangzhou:Levels and an assessment of human exposure[J]. Ecotox Environ Safe, 2019, 169:325-334. [76] YU Y J, LIN B G, CHEN X C, et al. Polybrominated diphenyl ethers in human serum, semen and indoor dust:Effects on hormones balance and semen quality[J]. Science of the Total Environment, 2019, 671:1017-1025. [77] KHAN M U, LI J, ZHANG G, et al. New insight into the levels, distribution and health risk diagnosis of indoor and outdoor dust-bound FRs in colder, rural and industrial zones of Pakistan[J]. Environ Pollut, 2016, 216:662-674. [78] YADAV I C, DEVI N L, SINGH V K, et al. Measurement of legacy and emerging flame retardants in indoor dust from a rural village (Kopawa) in Nepal:Implication for source apportionment and health risk assessment[J]. Ecotoxicol Environ Saf, 2019, 168:304-314. [79] QIAO L, ZHENG X B, ZHENG J, et al. Legacy and currently used organic contaminants in human hair and hand wipes of female e-waste dismantling workers and workplace dust in South China[J]. Environ Sci Technol, 2019, 53(5):2820-2829. [80] SUN J, XU Y, ZHOU H, et al. Levels, occurrence and human exposure to novel brominated flame retardants (NBFRs) and Dechlorane Plus (DP) in dust from different indoor environments in Hangzhou, China[J]. Sci Total Environ, 2018, 631/632:1212-1220. [81] LIU R, MABURY S A. Organophosphite antioxidants in indoor dust represent an indirect source of organophosphate esters[J]. Environ Sci Technol, 2019, 53(4):1805-1811. [82] BRITS M, BRANDSMA S H, ROHWER E R, et al. Brominated and organophosphorus flame retardants in South African indoor dust and cat hair[J]. Environ Pollut, 2019, 253:120-129. [83] KWEON D J, KIM M K, ZOH K D. Distribution of brominated flame retardants and phthalate esters in house dust in Korea[J]. Environmental Engineering Research, 2018, 23(4):354-363. [84] NIU D, QIU Y, LI L, et al. Occurrence of polybrominated diphenyl ethers in floor and elevated surface house dust from Shanghai, China[J]. Environ Sci Pollut Res Int, 2018, 25(18):18049-18058. [85] ALI N, EQANI S, ISMAIL I M I, et al. Brominated and organophosphate flame retardants in indoor dust of Jeddah, Kingdom of Saudi Arabia:Implications for human exposure[J]. Sci Total Environ, 2016, 569/570:269-277. [86] JILKOVA S, MELYMUK L, VOJTA S, et al. Small-scale spatial variability of flame retardants in indoor dust and implications for dust sampling[J]. Chemosphere, 2018, 206:132-141. [87] AL-OMRAN L S, HARRAD S. Influence of sampling approach on concentrations of legacy and "novel" brominated flame retardants in indoor dust[J]. Chemosphere, 2017, 178:51-58. [88] AL-OMRAN L S, HARRAD S. Distribution pattern of legacy and "novel" brominated flame retardants in different particle size fractions of indoor dust in Birmingham, United Kingdom[J]. Chemosphere, 2016, 157:124-131. [89] WEMKEN N, DRAGE D S, ABDALLAH M A E, et al. Concentrations of brominated flame retardants in indoor air and dust from ireland reveal elevated exposure to decabromodiphenyl ethane[J]. Environmental Science & Technology, 2019, 53(16):9826-9836. [90] JIN M, YIN J, ZHENG Y, et al. Pollution characteristics and sources of polybrominated diphenyl ethers in indoor air and dustfall measured in university laboratories in Hangzhou, China[J]. Sci Total Environ, 2018, 624:201-209. [91] KURT-KARAKUS P B, ALEGRIA H, JANTUNEN L, et al. Polybrominated diphenyl ethers (PBDEs) and alternative flame retardants (NFRs) in indoor and outdoor air and indoor dust from Istanbul-Turkey:Levels and an assessment of human exposure[J]. Atmospheric Pollution Research, 2017, 8(5):801-815. [92] OKONSKI K, MELYMUK L, KOHOUTEK J, et al. Hexabromocyclododecane:concentrations and isomer profiles from sources to environmental sinks[J]. Environ Sci Pollut Res Int, 2018, 25(36):36624-36635. [93] KHAN M U, BESIS A, LI J, et al. New insight into the distribution pattern, levels, and risk diagnosis of FRs in indoor and outdoor air at low- and high-altitude zones of Pakistan:Implications for sources and exposure[J]. Chemosphere, 2017, 184:1372-1387. [94] GOU Y Y, HSU Y C, CHAO H R, et al. Pollution characteristics and diurnal variations in polybrominated diphenyl ethers in indoor and outdoor air from vehicle dismantler factories in Southern Taiwan[J]. Aerosol and Air Quality Research, 2016, 16(8):1931-1941. [95] TAO F, SELLSTROM U, DE WIT C A. Organohalogenated flame retardants and organophosphate esters in office air and dust from Sweden[J]. Environ Sci Technol, 2019, 53(4):2124-2133. [96] BU Q, WU D, XIA J, et al. Polybrominated diphenyl ethers and novel brominated flame retardants in indoor dust of different microenvironments in Beijing, China[J]. Environ Int, 2019, 122:159-167. [97] CHEN Y, CAO Z, COVACI A, et al. Novel and legacy flame retardants in paired human fingernails and indoor dust samples[J]. Environ Int, 2019, 133(Pt B):105227. [98] CHEN M, PI L, LUO Y, et al. Grain size distribution and health risk assessment of metals in outdoor dust in Chengdu, Southwestern China[J]. Arch Environ Contam Toxicol, 2016, 70(3):534-543. [99] CHEN M, JIANG J, GAN Z, et al. Grain size distribution and exposure evaluation of organophosphorus and brominated flame retardants in indoor and outdoor dust and PM10 from Chengdu, China[J]. J Hazard Mater, 2019, 365:280-288. [100] TANG S, TAN H, LIU X, et al. Legacy and alternative flame retardants in house dust and hand wipes from South China[J]. Sci Total Environ, 2019, 656:1-8. [101] LARSSON K, DE WIT C A, SELLSTROM U, et al. Brominated flame retardants and organophosphate esters in preschool dust and children's hand wipes[J]. Environ Sci Technol, 2018, 52(8):4878-4888. [102] MELYMUK L, DIAMOND M L, RIDDELL N, et al. Challenges in the analysis of novel flame retardants in indoor dust:Results of the INTERFLAB 2 interlaboratory evaluation[J]. Environ Sci Technol, 2018, 52(16):9295-9303. [103] ARNOLD K, TEIXEIRA J P, MENDES A, et al. A pilot study on semivolatile organic compounds in senior care facilities:Implications for older adult exposures[J]. Environ Pollut, 2018, 240:908-915. [104] CRISTALE J, ARAGAO BELE T G, LACORTE S, et al. Occurrence and human exposure to brominated and organophosphorus flame retardants via indoor dust in a Brazilian city[J]. Environ Pollut, 2018, 237:695-703. [105] WANG J, WANG Y, SHI Z, et al. Legacy and novel brominated flame retardants in indoor dust from Beijing, China:Occurrence, human exposure assessment and evidence for PBDEs replacement[J]. Sci Total Environ, 2018, 618:48-59. [106] YU Y J, LIN B G, LIANG W B, et al. Associations between PBDEs exposure from house dust and human semen quality at an e-waste areas in South China-A pilot study[J]. Chemosphere, 2018, 198:266-273. [107] IWEGBUE C M A, EYENGHO S B, EGOBUEZE F E, et al. Polybrominated diphenyl ethers and polychlorinated biphenyls in indoor dust from electronic repair workshops in southern Nigeria:Implications for onsite human exposure[J]. Science of The Total Environment, 2019, 671:914-927. [108] NKABINDE S N, OKONKWO J O, OLUKUNLE O I, et al. Determination of legacy and novel brominated flame retardants in dust from end of life office equipment and furniture from Pretoria, South Africa[J]. Sci Total Environ, 2018, 622/623:275-281. [109] XIONG P, YAN X, ZHU Q, et al. A review of environmental occurrence, fate, and toxicity of novel brominated flame retardants[J]. Environ Sci Technol, 2019, 53(23):13551-13569. [110] TAKIGAMI H, SUZUKI G, HIRAI Y, et al. Brominated flame retardants and other polyhalogenated compounds in indoor air and dust from two houses in Japan[J]. Chemosphere, 2009, 76(2):270-277. [111] FUNG A G, YAMAGUCHI M S, MCCARTNEY M M, et al. SPME-based mobile field device for active sampling of volatiles[J]. Microchem J, 2019, 146:407-413. [112] VAN DROOGE B L, GRIMALT J O, BOOIJ K, et al. Passive sampling of atmospheric organochlorine compounds by SPMDs in a remote high mountain area[J]. Atmospheric Environment, 2005, 39(28):5195-5204. -
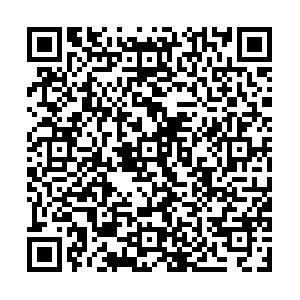
计量
- 文章访问数: 2809
- HTML全文浏览数: 2809
- PDF下载数: 53
- 施引文献: 0