根际微生物增强宿主植物耐铬能力生理机制研究进展
The Role of Rhizosphere Microorganisms in Enhancing Chromium Tolerance of Host Plants
-
摘要: 植物根际有大量微生物,其中一部分微生物,如根际促生菌、丛枝菌根真菌、非麦角属内生真菌和外生菌根真菌,在改善宿主营养状况,缓解宿主由于旱涝、盐分和重金属等环境胁迫导致的危害中起到重要作用。笔者将以这4种根际微生物为例,综述它们提高宿主植物耐铬性的内在机制,如通过促进宿主生长、降低根际土壤中铬的有效性、降低铬从根系向叶片的转运以及利用自身组织固持铬等,直接或间接地提高植物对铬的耐受能力,展现出多样且互有差异的功能。同时,笔者提出了铬在根际微生物与宿主的共生体系内转运和解毒行为的分子和生理机制上的不足,并对未来根际微生物互作的研究内容提出了展望。Abstract: There are ubiquitous microorganisms in the rhizosphere forming symbiosis with host plants. Some of them, including plant growth promoting rhizobacteria, arbuscular mycorrhizal fungi, non-clavicipitaceous endophytes and ectomycorrhizal fungi, play important roles in improving plant nutrition status and alleviating the damage caused by drought, flooding, salinity and heavy metal stress. This paper reviewed the underlying mechanisms of enhancing plant chromium (Cr) tolerance by these four groups of rhizosphere microorganisms. As a whole, they can directly or indirectly improve plant Cr tolerance via various and different functions including promoting plant growth, reducing the Cr bioavailability, decreasing the translocation of metal from roots to shoots, stabilizing metal by fungal structures and so on. However, the physiological and molecular mechanisms underlying Cr behavior and detoxification in rhizosphere remain largely unknown. It's also of great importance to clarify the effect of interaction networks between the rhizosphere microorganisms.
-
Key words:
- chromium stress /
- rhizosphere microorganisms /
- tolerance mechanism /
- interaction networks
-
复方益母草胶囊是由益母草、当归和熟地3味药材经过一定的工序加工制成的复方制剂,该胶囊主要药味益母草中含有生物碱类、黄酮类、二萜类、苷类、脂肪酸类、挥发油类等成分[1]. 生物碱类盐酸水苏碱是益母草的主要药效成分[2]. 盐酸水苏碱是季胺碱,极性强,无共轭结构,紫外吸收弱,属于末端吸收. 高效液相色谱法是盐酸水苏碱目前最为普遍的检测方法,盐酸水苏碱常用的检测器有紫外检测器 (DAD等) [3]、质谱检测器(MS)[4]、示差检测器(RID)和蒸发光散射检测器 (ELSD) [5]等,目前尚未报道电雾式检测器(CAD)被用于盐酸水苏碱的定量分析. 用紫外检测器测定盐酸水苏碱,存在方法重复性差、溶剂末端吸收干扰、梯度洗脱时容易出现基线漂移检测不稳定等局限性[6];依据盐酸水苏碱的结构中有旋光性的特点,采用RID检测器测定其含量,无需特殊样品处理方法就有很好的峰形,但专属性不强、灵敏度较低,且不能使用梯度洗脱分离效果不佳,应用相对较少[7-8];ELSD检测器是目前定量分析盐酸水苏碱最常用的检测器,据报道其灵敏度也较低[9]. 电雾式检测器(CAD)是一种质量相关的通用型检测器,其检测信号不依赖于被测物质的化学结构,更适用于无紫外吸收或只有较弱紫外吸收成分的定量分析.
本研究利用Thermo-fisher AcclaimTM Mixed-Mode WAX-1色谱柱结合HPLC-CAD法分离测定复方益母草胶囊中主要成分盐酸水苏碱含量,探讨该方法对比现行《中国药典》(2020版)一部中ELSD测定盐酸水苏碱的优势,为其质量评价和标准制定提供理论依据,同时为未来推广到其它含益母草类制剂中使用提供科学参考,具有现实应用价值,且目前缺少相关报道.
1. 实验部分
1.1 仪器与试剂
试剂:甲酸分析纯(纯度99.9%)(天津市科密欧化学试剂有限公司);甲酸铵质谱级(纯度99%)(Roe Scientific Inc公司);无水乙醇分析纯(天津市富宇精细化工有限公司);甲醇HPLC级(上海星可高纯溶剂有限公司);乙腈HPLC级(上海星可高纯溶剂有限公司);屈臣氏饮用水(广州屈臣氏食品饮料有限公司);盐酸水苏碱对照品(纯度98.91%)(成都普思生物科技股份有限公司).
液相色谱(美国赛默飞公司),Vanquish Core系列双三元泵:VC-P33-A-01;自动进样器:VC-A12-A-02;柱温箱:Column compartment VC-C10-A-03;可变波长检测器:Variable Wavelength Detector VC-D40-A-01;CAD检测器:Charged Aerosol Detector H VH-D20-A;变色龙色谱管理软件 Chromeleon CDS 7.3; Waters Acquity超高效液相色谱仪ELS Detector检测器;电子天平 YP30002(上海佑科仪器仪表公司);分析天平 B931029762(北京艾斯瑞克商贸公司);超声仪 KQ-250DB(昆山市超声仪器公司)。
色谱柱:AccucoreTM-Amide-HILIC(150 mm × 4.6 mm,5 µm, Thermo-Fisher公司);AcclaimTM Mixed-Mode WAX-1(250 mm × 4.6 mm,5 µm, Thermo-Fisher公司); AcclaimTM Mixed-Mode WCX-1(250 mm × 4.6 mm,5 μm, Thermo-Fisher 公司);Ascentis Express OH5 (150 mm × 4.6 mm,2.7 µm, 美国merck公司);Polar-Phenyl (250 mm × 4.6 mm,5 µm, 济南赛畅科学仪器有限公司);ShimNex HE Amide (250 mm × 4.6 mm,5 µm ,岛津中国公司);Poroshell 120 HILIC-Z (150 mm × 4.6 mm,2.7 µm, 安捷伦公司);Venusil HILIC (250 mm × 4.6 mm,5 µm,Agelag公司);XBridge BEH Amide (150 mm × 4.6 mm,5 µm Waters公司);60-5-HILIC-D (250 mm × 4.6 mm,5 µm Kromasil公司); XBridge Amide (250 mm × 4.6 mm,5 µm Waters公司).
1.2 溶液配制
对照品溶液 取盐酸水苏碱对照品适量,精密测定,加入70%乙腈(乙醇)制成每1mL含0.5 mg的溶液,即得.
供试品溶液 按2020版《中国药典》方法制备,取装量差异项下的复方益母草胶囊内容物,混匀,研细,取约0.5 g,精密称定,置具塞锥形瓶中,精密加入70% 乙腈(乙醇)25 mL,称定重量,加热回流2 h,放冷,再称定重量,用70%乙腈(乙醇)补足减失的重量,摇匀,滤过,取续滤液,即得.
阴性对照的制备:按照复方益母草胶囊处方药物组成,不加益母草和辅料,同供试品制备方法.
2. 结果与讨论
2.1 检测器选择
精密量取盐酸水苏碱对照品储备液1 mL,分别置于5、10、20、50、100 mL容量瓶中,至刻度. 再精密量取1 mL上述50 mL容量瓶溶液置于10 mL、25 mL容量瓶中,加70%乙醇置刻度,摇匀. 按照《中国药典》(2020版)一部复方益母草胶囊方法,采用AccucoreTM-150-Amide-HILIC (150 mm × 4.6 mm, 5 µm)色谱柱,以乙腈-0.2%冰醋酸溶液(80∶20)为流动相,进样量5 μL,分别用ELSD和CAD检测器测定盐酸水苏碱的信噪比,进行灵敏度对比. 按信噪比3∶1及10∶1计算,盐酸水苏碱的检测限(LOD)和定量限(LOQ)结果显示,CAD检测限是ELSD的34倍,定量限是37倍,表明CAD有更好的灵敏度.
2.2 色谱柱选择
取盐酸水苏碱标准品适量,分别采用10种不同厂家的色谱柱进行测定,其中Poroshell 120 HILIC-Z和AcclaimTM Mixed-Mode WAX-1色谱柱对复方益母草中盐酸水苏碱分离效果最好,前者理论塔板数高达26430,后者理论塔板数18413,且前后无干扰峰,因此本研究最终选择AcclaimTM Mixed-Mode WAX-1柱子作为盐酸水苏碱的定量分析.
2.3 检测方法研究
盐酸水苏碱是最简单的吡咯生物碱,其分子结构含有季铵根和羧基,极性较强。实验室尝试了 Acclaim Mixed-Mode WAX-1 和 WCX-1 两款色谱柱的 RP+IEX 保留模式,保留均较弱。其中 Acclaim Mixed-Mode WAX-1 色谱柱键合相为带有叔胺末端的疏水烷基链键合硅胶,除去反相保留(RP)和弱阴离子交换(WAX),还可提供亲水作用(HILIC)保留机制。经试验确定采用 Acclaim Mixed-Mode WAX-1 色谱柱的 HILIC 模式进行盐酸水苏碱的分离保留效果优异,因而流动相有机相优先选择乙腈。由于 Acclaim Mixed-Mode WAX-1 色谱柱必须始终使用含有缓冲盐的流动相进行活化、分析或保存,在配合 CAD 使用时,须使用挥发性缓冲盐,流动相水相可选用甲酸铵或乙酸铵。
除了色谱柱和流动相的选择外,供试品溶液的制备也需要重点考察。取复方益母草胶囊数粒去壳,将内容物混匀研细,取0.2 g,精密称定,置具塞三角瓶中,分别精密加入30%、50%、70%的甲醇,30%、50%、70%的乙醇和30%、50%、70%的乙腈,超声提取40 min(功率250 W,频率40 kHz),冷却至室温,摇匀. 离心5 min(5000 r·min−1)。转移至25 mL容量瓶并定容至刻度,经0.45 μm微孔滤膜过滤,取续滤液,即得. 进样检测,计算各提取溶剂下盐酸水苏碱的相对含量. 3种不同浓度的乙腈对盐酸水苏碱成分提取效果最好,在 HILIC 模式下,流动相通常推荐使用非质子溶剂乙腈,并且初始流动相为较高比例的乙腈,当对照品或供试品溶液乙腈比例较低时,进样后容易形成溶剂效应,影响水苏碱的峰形和响应。试验表明,采用 70% 乙腈水提取效果较好,因此本研究最终选择70%乙腈作为提取溶剂.
最终确定以CAD为检测器,采用AcclaimTM Mixed-Mode WAX-1(250 mm×4.6 mm,5 μm)柱,以20 mmol·L-1 甲酸铵 (甲酸调节 pH至 4.0) (A)-乙腈(B)为流动相,梯度洗脱:0—8 min,10%A;8—12 min,10%—50%A;12—15 min,50%A;15—20 min,50%—10%A. 柱温30 ℃,蒸发温度35 ℃;采集频率 5 Hz,过滤常数 3.6 s;进样体积5 μL. 该色谱条件通过系统考察得到较好的分离效果.
2.4 方法学验证
分别取盐酸水苏碱标准对照品溶液,供试品溶液,缺益母草阴性对照液适量,注入高效液相色谱仪,按2.3节色谱条件进样测定. 供试品色谱图中出现与标准对照品中保留时间相同的峰,且理论塔板数不低于18000,缺益母草阴性对照色谱图中显示阴性样品无干扰,表明该方法专属性较好(图1).
取盐酸水苏碱对照品溶液,按2.3节色谱条件连续进样6次,记录峰面积,结果峰面积RSD为1.13%. 按上述样品制备方法,制备供试品溶液,分别于0、2、6、10、24 h进样,RSD为1.93%,表明供试品在24 h内稳定性良好. 取同一批号样品(批号:20220301),按2.3节方法平行制备6份供试品溶液,在2.3节色谱条件进样分析,样品中盐酸水苏碱平均含量33.4 mg·g−1,RSD为1.38%.
采用加样回收法,精密称取0.1 g已知含量的复方益母草胶囊样品8份,分别加入适量的盐酸水苏碱标准品,按2.3节供试品制备方式制备供试品. 计算加样回收率在95.70%—99.20%,平均回收率97.37%,RSD为1.14%(表1).
表 1 盐酸水苏碱回收率(n=2)Table 1. Recovery rate of stachydrine hydrochloride(n=2)序号 取样量/g 样品含有量/mg 加标量/mg 测得量/mg 回收率/% 平均回收率/% 相对标准偏差/% 1 0.1042 3.4821 3.3420 6.7972 99.1953 97.37 1.14 2 0.1095 3.5389 3.3420 6.7904 97.2901 3 0.1079 3.6058 3.3420 6.8040 95.6998 4 0.1031 3.4454 3.3420 6.6717 96.5381 5 0.1096 3.6626 3.3420 6.9328 97.8527 6 0.1062 3.5489 3.3420 6.8387 98.4361 7 0.1040 3.4754 3.3420 6.7093 96.7638 8 0.1047 3.4988 3.3420 6.7481 97.2262 精密称量盐酸水苏碱对照品9.30 g于20 mL容量瓶中,加70%乙腈水溶液逐级稀释,制成盐酸水苏碱浓度分别为0.465、0.279、0.186、0.093、0.047、0.019 mg·mL−1,按2.3项下色谱条件下进样分析,记录相应峰面积. 以待测组分质量浓度(X)为横坐标,以峰面积(Y)为纵坐标,对一次曲线和二次曲线进行了比较,一次曲线函数为Y=52.989X+1.5521,r2为0.9862,二次曲线函数为Y=-54.114X2+78.28X+0.4612,r2为0.9995,二次曲线拟合较好,故选二次曲线进行计算.
2.5 复方益母草胶囊含量测定
依据前述盐酸水苏碱含量测定方法,分别对随机选取的同批次和不同批次(5批)复方益母草胶囊中盐酸水苏碱含量进行测定,分别计算其批内(PA)和批间(PB)盐酸水苏碱含量的差异值.结果表明,复方益母草胶囊批内差异PA值为6.3%,反映该厂家与益母草相关的生产工艺较稳定. 批间一致性差异较大,每粒含量12.00—16.14 mg不等,PB值为28.98%,推测可能是益母草原料质量差异造成.
3. 结论
通过对比10种亲水型作用色谱柱(HILIC),最终确定利用Acclaim Mixed-Mode WAX-1色谱柱键合相的叔胺官能团,在 HILIC 模式下对水苏碱的强保留能力,可有效与供试品杂质峰分离,避免基质干扰。采用乙腈-20 mmol·L−1 甲酸铵缓冲液 (pH=4.0) = 90:10作为初始流动相进行梯度洗脱,配合CAD检测器测定盐酸水苏碱含量, 结果显示CAD具有较高的灵敏度,为定量分析盐酸水苏碱提供了新方法新参考. 本研究所建立用于测定盐酸水苏碱HPLC-CAD方法,专属性强、重复性好、灵敏度高,为复方益母草胶囊及相关制剂中盐酸水苏碱的质量检测提供可行方案.
-
Pradas del Real A E, García-Gonzalo P, Lobo M C, et al. Chromium speciation modifies root exudation in two genotypes of Silene vulgaris[J]. Environmental and Experimental Botany, 2014, 107:1-6 Kotaś J, Stasicka Z. Chromium occurrence in the environment and methods of its speciation[J]. Environmental Pollution, 2000, 107(3):263-283 Mohanty M, Patra H K. Attenuation of chromium toxicity by bioremediation technology[J]. Reviews of Environmental Contamination and Toxicology, 2011, 210:1-34 Kimbrough D E, Cohen Y, Winer A M, et al. A critical assessment of chromium in the environment[J]. Critical Reviews in Environmental Science and Technology, 1999, 29(1):1-46 Fendorf S E. Surface reactions of chromium in soils and waters[J]. Geoderma, 1995, 67(1-2):55-71 Panda S K, Choudhury S. Chromium stress in plants[J]. Brazilian Journal of Plant Physiology, 2005, 17(1):95-102 Losi M E, Amrhein C, Frankenberger W T Jr. Environmental biochemistry of chromium[J]. Reviews of Environmental Contamination and Toxicology, 1994, 136:91-121 Cheung K H, Gu J D. Mechanism of hexavalent chromium detoxification by microorganisms and bioremediation application potential:A review[J]. International Biodeterioration & Biodegradation, 2007, 59(1):8-15 Singh H P, Mahajan P, Kaur S, et al. Chromium toxicity and tolerance in plants[J]. Environmental Chemistry Letters, 2013, 11(3):229-254 Rai V, Vajpayee P, Singh S N, et al. Effect of chromium accumulation on photosynthetic pigments, oxidative stress defense system, nitrate reduction, proline level and eugenol content of Ocimum tenuiflorum L.[J]. Plant Science, 2004, 167(5):1159-1169 Poschenrieder C, Gunse B, Barcelo J. Chromium-induced inhibition of ethylene evolution in bean (Phaseolus vulgaris) leaves[J]. Physiologia Plantarum, 1993, 89(2):404-408 张黛静, 姜丽娜, 邵云, 等. 铬胁迫下不同品种小麦萌发和内源激素的变化[J]. 应用与环境生物学报, 2009, 15(5):602-605 Zhang D J, Jiang L N, Shao Y, et al. Variations in germination and endogenous hormone contents of wheat cultivars under Cr stress[J]. Chinese Journal of Applied & Environmental Biology, 2009, 15(5):602-605(in Chinese)
Montes-Holguin M O, Peralta-Videa J R, Meitzner G, et al. Biochemical and spectroscopic studies of the response of Convolvulus arvensis L. to chromium(Ⅲ) and chromium(Ⅵ) stress[J]. Environmental Toxicology and Chemistry, 2006, 25(1):220-226 Pandey V, Dixit V, Shyam R. Chromium(Ⅵ) induced changes in growth and root plasma membrane redox activities in pea plants[J]. Protoplasma, 2009, 235(1-4):49-55 Vernay P, Gauthier-Moussard C, Hitmi A. Interaction of bioaccumulation of heavy metal chromium with water relation, mineral nutrition and photosynthesis in developed leaves of Lolium perenne L.[J]. Chemosphere, 2007, 68(8):1563-1575 Subrahmanyam D. Effects of chromium toxicity on leaf photosynthetic characteristics and oxidative changes in wheat (Triticum aestivum L.)[J]. Photosynthetica, 2008, 46(3):339-345 Schiavon M, Pilon-Smits E A, Wirtz M, et al. Interactions between chromium and sulfur metabolism in Brassica juncea[J]. Journal of Environmental Quality, 2008, 37(4):1536-1545 Gopal R, Rizvi A H, Nautiyal N. Chromium alters iron nutrition and water relations of spinach[J]. Journal of Plant Nutrition, 2009, 32(9):1551-1559 Vajpayee P, Tripathi R D, Rai U N, et al. Chromium(Ⅵ) accumulation reduces chlorophyll biosynthesis, nitrate reductase activity and protein content in Nymphaea alba L.[J]. Chemosphere, 2000, 41(7):1075-1082 Shanker A K, Cervantes C, Loza-Tavera H, et al. Chromium toxicity in plants[J]. Environment International, 2005, 31(5):739-753 Duman F, Koca F D, Sahan S. Antagonist effects of sodium chloride on the biological responses of an aquatic plant (Ceratophyllum demersum L.) exposed to hexavalent chromium[J]. Water, Air, & Soil Pollution, 2014, 225(2):1-12 Pereira M, Bartolomé M C, Sánchez-Fortún S. Bioadsorption and bioaccumulation of chromium trivalent in Cr(Ⅲ)-tolerant microalgae:A mechanisms for chromium resistance[J]. Chemosphere, 2013, 93(6):1057-1063 Chandra P, Kulshreshtha K. Chromium accumulation and toxicity in aquatic vascular plants[J]. The Botanical Review, 2004, 70(3):313-327 Choo T P, Lee C K, Low K S, et al. Accumulation of chromium(Ⅵ) from aqueous solutions using water lilies (Nymphaea spontanea)[J]. Chemosphere, 2006, 62(6):961-967 Ganesh K S, Baskaran L, Rajasekaran S, et al. Chromium stress induced alterations in biochemical and enzyme metabolism in aquatic and terrestrial plants[J]. Colloids and Surfaces B, Biointerfaces, 2008, 63(2):159-163 Sangwan P, Kumar V, Joshi U N. Effect of chromium(Ⅵ) toxicity on enzymes of nitrogen metabolism in clusterbean (Cyamopsis tetragonoloba L.)[J]. Enzyme Research, 2014, 2014:784036 Shukla O P, Dubey S, Rai U N. Preferential accumulation of cadmium and chromium:Toxicity in Bacopa monnieri L. under mixed metal treatments[J]. Bulletin of Environmental Contamination and Toxicology, 2007, 78(3-4):252-257 Kundu D, Dey S, Raychaudhuri S S. Chromium(Ⅵ)-induced stress response in the plant Plantago ovata Forsk in vitro[J]. Genes and Environment, 2018, 40:21 Narendrula-Kotha R, Theriault G, Mehes-Smith M, et al. Metal toxicity and resistance in plants and microorganisms in terrestrial ecosystems[J]. Reviews of Environmental Contamination and Toxicology, 2020, 249:1-27 Abdu N, Abdullahi A A, Abdulkadir A. Heavy metals and soil microbes[J]. Environmental Chemistry Letters, 2017, 15(1):65-84 Kong Z Y, Glick B R. The role of plant growth-promoting bacteria in metal phytoremediation[J]. Advances in Microbial Physiology, 2017, 71:97-132 Glick B R. Plant growth-promoting bacteria:Mechanisms and applications[J]. Scientifica, 2012, 2012:963401 Ma Y, Prasad M N, Rajkumar M, et al. Plant growth promoting rhizobacteria and endophytes accelerate phytoremediation of metalliferous soils[J]. Biotechnology Advances, 2011, 29(2):248-258 Pandey P, Irulappan V, Bagavathiannan M V, et al. Impact of combined abiotic and biotic stresses on plant growth and avenues for crop improvement by exploiting physio-morphological traits[J]. Frontiers in Plant Science, 2017, 8:537 Benizri E, Baudoin E, Guckert A. Root colonization by inoculated plant growth-promoting rhizobacteria[J]. Biocontrol Science and Technology, 2001, 11(5):557-574 Ahemad M. Enhancing phytoremediation of chromium-stressed soils through plant-growth-promoting bacteria[J]. Journal of Genetic Engineering & Biotechnology, 2015, 13(1):51-58 Gupta P, Kumar V, Usmani Z, et al. A comparative evaluation towards the potential of Klebsiella sp. and Enterobacter sp. in plant growth promotion, oxidative stress tolerance and chromium uptake in Helianthus annuus (L.)[J]. Journal of Hazardous Materials, 2019, 377:391-398 Somers E, Vanderleyden J, Srinivasan M. Rhizosphere bacterial signalling:A love parade beneath our feet[J]. Critical Reviews in Microbiology, 2004, 30(4):205-240 Indiragandhi P, Anandham R, Madhaiyan M, et al. Characterization of plant growth-promoting traits of bacteria isolated from larval guts of diamondback moth Plutella xylostella (Lepidoptera:Plutellidae)[J]. Current Microbiology, 2008, 56(4):327-333 Neubauer U, Furrer G, Kayser A, et al. Siderophores, NTA, and citrate:Potential soil amendments to enhance heavy metal mobility in phytoremediation[J]. International Journal of Phytoremediation, 2000, 2(4):353-368 Rajkumar M, Nagendran R, Lee K J, et al. Influence of plant growth promoting bacteria and Cr6+ on the growth of Indian mustard[J]. Chemosphere, 2006, 62(5):741-748 Belimov A A, Hontzeas N, Safronova V I, et al. Cadmium-tolerant plant growth-promoting bacteria associated with the roots of Indian mustard (Brassica juncea L. Czern)[J]. Soil Biology and Biochemistry, 2005, 37(2):241-250 Gupta P, Rani R, Chandra A, et al. Potential applications of Pseudomonas sp. (strain CPSB21) to ameliorate Cr6+ stress and phytoremediation of tannery effluent contaminated agricultural soils[J]. Scientific Reports, 2018, 8(1):4860 Hemambika B, Balasubramanian V, Rajesh Kannan V, et al. Screening of chromium-resistant bacteria for plant growth-promoting activities[J]. Soil and Sediment Contamination, 2013, 22(7):717-736 Khan N, Mishra A, Chauhan P S, et al. Paenibacillus lentimorbus enhances growth of chickpea (Cicer arietinum L.) in chromium-amended soil[J]. Antonie Van Leeuwenhoek, 2012, 101(2):453-459 Morel M A, Ubalde M C, Braña V, et al. Delftia sp. JD2:A potential Cr(Ⅵ)-reducing agent with plant growth-promoting activity[J]. Archives of Microbiology, 2011, 193(1):63-68 Wani P A, Khan M S, Zaidi A. Chromium reduction, plant growth-promoting potentials, and metal solubilizatrion by Bacillus sp. isolated from alluvial soil[J]. Current Microbiology, 2007, 54(3):237-243 Vosatka M, Gryndler M, Prikryl Z. Effect of the rhizosphere bacterium Pseudomonas putida, arbuscular mycorrhizal fungi and substrate composition on the growth of strawberry[J]. Agronomie, 1992, 12(10):859-863 Todeschini V, AitLahmidi N, Mazzucco E, et al. Impact of beneficial microorganisms on strawberry growth, fruit production, nutritional quality, and volatilome[J]. Frontiers in Plant Science, 2018, 9:1611 曾加会, 李元媛, 阮迪申, 等. 植物根际促生菌及丛枝菌根真菌协助植物修复重金属污染土壤的机制[J]. 微生物学通报, 2017, 44(5):1214-1221 Zeng J H, Li Y Y, Ruan D S, et al. Phytoremediation of heavy metal contaminated soils by plant growth-promoting rhizobacteria and arbuscular mycorrhizal fungi[J]. Microbiology China, 2017, 44(5):1214-1221(in Chinese)
Islam F, Yasmeen T, Arif M S, et al. Combined ability of chromium (Cr) tolerant plant growth promoting bacteria (PGPB) and salicylic acid (SA) in attenuation of chromium stress in maize plants[J]. Plant Physiology and Biochemistry, 2016, 108:456-467 Xu Z Y, Ban Y H, Jiang Y H, et al. Arbuscular mycorrhizal fungi in wetland habitats and their application in constructed wetland:A review[J]. Pedosphere, 2016, 26(5):592-617 Lenoir I, Fontaine J, Lounès-Hadj Sahraoui A. Arbuscular mycorrhizal fungal responses to abiotic stresses:A review[J]. Phytochemistry, 2016, 123:4-15 Leyval C, Turnau K, Haselwandter K. Effect of heavy metal pollution on mycorrhizal colonization and function:Physiological, ecological and applied aspects[J]. Mycorrhiza, 1997, 7(3):139-153 Ferrol N, Tamayo E, Vargas P. The heavy metal paradox in arbuscular mycorrhizas:From mechanisms to biotechnological applications[J]. Journal of Experimental Botany, 2016, 67(22):6253-6265 Nakatani A S, Mescolotti D L C, Nogueira M A, et al. Dosage-dependent shift in the spore community of arbuscular mycorrhizal fungi following application of tannery sludge[J]. Mycorrhiza, 2011, 21(6):515-522 Coughlan A P, Dalpe Y, Lapointe L, et al. Soil pH-induced changes in root colonization, diversity, and reproduction of symbiotic arbuscular mycorrhizal fungi from healthy and declining maple forests[J]. Canadian Journal of Forest Research, 2000, 30(10):1543-1554 Wu S L, Hu Y J, Zhang X, et al. Chromium detoxification in arbuscular mycorrhizal symbiosis mediated by sulfur uptake and metabolism[J]. Environmental and Experimental Botany, 2018, 147:43-52 Singh S, Parihar P, Singh R, et al. Heavy metal tolerance in plants:Role of transcriptomics, proteomics, metabolomics, and ionomics[J]. Frontiers in Plant Science, 2015, 6:1143 Wu S L, Zhang X, Huang L B, et al. Arbuscular mycorrhiza and plant chromium tolerance[J]. Soil Ecology Letters, 2019, 1(3-4):94-104 Wang W X, Shi J C, Xie Q J, et al. Nutrient exchange and regulation in arbuscular mycorrhizal symbiosis[J]. Molecular Plant, 2017, 10(9):1147-1158 冯欢, 蒙盼盼, 豆青, 等. 菌根真菌与植物共生营养交换机制研究进展[J]. 应用生态学报, 2019, 30(10):3596-3604 Feng H, Meng P P, Dou Q, et al. Advances in mechanisms of nutrient exchange between mycorrhizal fungi and host plants[J]. Chinese Journal of Applied Ecology, 2019, 30(10):3596-3604(in Chinese)
Tang N W, San Clemente H, Roy S, et al. A survey of the gene repertoire of Gigaspora rosea unravels conserved features among glomeromycota for obligate biotrophy[J]. Frontiers in Microbiology, 2016, 7:233 Rausch C, Bucher M. Molecular mechanisms of phosphate transport in plants[J]. Planta, 2002, 216(1):23-37 Bucher M. Functional biology of plant phosphate uptake at root and mycorrhiza interfaces[J]. The New Phytologist, 2007, 173(1):11-26 Müller L M, Harrison M J. Phytohormones, miRNAs, and peptide signals integrate plant phosphorus status with arbuscular mycorrhizal symbiosis[J]. Current Opinion in Plant Biology, 2019, 50:132-139 Gil-Cardeza M L, Calonne-Salmon M, Gómez E, et al. Short-term chromium(Ⅵ) exposure increases phosphorus uptake by the extraradical mycelium of the arbuscular mycorrhizal fungus Rhizophagus irregularis MUCL 41833[J]. Chemosphere, 2017, 187:27-34 Benedetto A, Magurno F, Bonfante P, et al. Expression profiles of a phosphate transporter gene (GmosPT) from the endomycorrhizal fungus Glomus mosseae[J]. Mycorrhiza, 2005, 15(8):620-627 Xie X N, Lin H, Peng X W, et al. Arbuscular mycorrhizal symbiosis requires a phosphate transceptor in the Gigaspora margarita fungal symbiont[J]. Molecular Plant, 2016, 9(12):1583-1608 de Plassard C, Becquer A, Garcia K. Phosphorus transport in mycorrhiza:How far are we?[J]. Trends in Plant Science, 2019, 24(9):794-801 Wu S L, Chen B D, Sun Y Q, et al. Chromium resistance of dandelion (Taraxacum platypecidum Diels.) and bermudagrass (Cynodon dactylon Pers.) is enhanced by arbuscular mycorrhiza in Cr(Ⅵ)-contaminated soils[J]. Environmental Toxicology and Chemistry, 2014, 33(9):2105-2113 陈保冬, 张莘, 伍松林, 等. 丛枝菌根影响土壤-植物系统中重金属迁移转化和累积过程的机制及其生态应用[J]. 岩矿测试, 2019, 38(1):1-25 Chen B D, Zhang X, Wu S L, et al. The role of arbuscular mycorrhizal fungi in heavy metal translocation, transformation and accumulation in the soil-plant continuum:Underlying mechanisms and ecological implications[J]. Rock and Mineral Analysis, 2019, 38(1):1-25(in Chinese)
Costa R C, Moura F C, Oliveira P E, et al. Controlled reduction of red mud waste to produce active systems for environmental applications:Heterogeneous Fenton reaction and reduction of Cr(Ⅵ)[J]. Chemosphere, 2010, 78(9):1116-1120 Anjum N A, Ahmad I, Mohmood I, et al. Modulation of glutathione and its related enzymes in plants' responses to toxic metals and metalloids-A review[J]. Environmental and Experimental Botany, 2012, 75:307-324 Foyer C H, Noctor G. Oxidant and antioxidant signalling in plants:A re-evaluation of the concept of oxidative stress in a physiological context[J]. Plant, Cell and Environment, 2005, 28(8):1056-1071 沈亚琴, 魏源, 陈志鹏, 等. 锑胁迫下丛枝菌根真菌对玉米生长与锑吸收及抗氧化酶的影响[J]. 环境科学研究, 2017, 30(5):712-719 Shen Y Q, Wei Y, Chen Z P, et al. Effects of arbuscular mycorrhizal fungi on growth, antimony uptake and antioxidant enzymes of maize under antimony stress[J]. Research of Environmental Sciences, 2017, 30(5):712-719(in Chinese)
Sharma V, Parmar P, Kumari N. Differential cadmium stress tolerance in wheat genotypes under mycorrhizal association[J]. Journal of Plant Nutrition, 2016, 39(14):2025-2036 Pallara G, Todeschini V, Lingua G, et al. Transcript analysis of stress defence genes in a white poplar clone inoculated with the arbuscular mycorrhizal fungus Glomus mosseae and grown on a polluted soil[J]. Plant Physiology and Biochemistry, 2013, 63:131-139 Gil-Cardeza M L, Ferri A, Cornejo P, et al. Distribution of chromium species in a Cr-polluted soil:Presence of Cr(Ⅲ) in glomalin related protein fraction[J]. Science of the Total Environment, 2014, 493:828-833 Arias J A, Peralta-Videa J R, Ellzey J T, et al. Plant growth and metal distribution in tissues of Prosopis juliflora-velutina grown on chromium contaminated soil in the presence of Glomus deserticola[J]. Environmental Science & Technology, 2010, 44(19):7272-7279 Wu S L, Zhang X, Chen B D, et al. Chromium immobilization by extraradical mycelium of arbuscular mycorrhiza contributes to plant chromium tolerance[J]. Environmental and Experimental Botany, 2016, 122:10-18 Wu S L, Zhang X, Sun Y Q, et al. Chromium immobilization by extra- and intraradical fungal structures of arbuscular mycorrhizal symbioses[J]. Journal of Hazardous Materials, 2016, 316:34-42 Holland S L, Avery S V. Chromate toxicity and the role of sulfur[J]. Metallomics:Integrated Biometal Science, 2011, 3(11):1119-1123 Wu S L, Zhang X, Chen B D, et al. Chromium immobilization by extraradical mycelium of arbuscular mycorrhiza contributes to plant chromium tolerance[J]. Environmental and Experimental Botany, 2016, 122:10-18 Wu S L, Zhang X, Sun Y Q, et al. Transformation and immobilization of chromium by arbuscular mycorrhizal fungi as revealed by SEM-EDS, TEM-EDS, and XAFS[J]. Environmental Science & Technology, 2015, 49(24):14036-14047 Schulz B, Boyle C, Draeger S, et al. Endophytic fungi:A source of novel biologically active secondary metabolites[J]. Mycological Research, 2002, 106(9):996-1004 Li H Y, Wei D Q, Shen M, et al. Endophytes and their role in phytoremediation[J]. Fungal Diversity, 2012, 54(1):11-18 Rozpądek P, Domka A, Ważny R, et al. How does the endophytic fungus Mucor sp. improve Arabidopsis arenosa vegetation in the degraded environment of a mine dump?[J]. Environmental and Experimental Botany, 2018, 147:31-42 Ent A, Baker A J M, Reeves R D, et al. Hyperaccumulators of metal and metalloid trace elements:Facts and fiction[J]. Plant and Soil, 2013, 362(1-2):319-334 Domka A M, Rozpaądek P, Turnau K. Are fungal endophytes merely mycorrhizal copycats? The role of fungal endophytes in the adaptation of plants to metal toxicity[J]. Frontiers in Microbiology, 2019, 10:371 Hardoim P R, van Overbeek L S, Berg G, et al. The hidden world within plants:Ecological and evolutionary considerations for defining functioning of microbial endophytes[J]. Microbiology and Molecular Biology Reviews, 2015, 79(3):293-320 Atsatt P R, Whiteside M D. Novel symbiotic protoplasts formed by endophytic fungi explain their hidden existence, lifestyle switching, and diversity within the plant kingdom[J]. PLoS One, 2014, 9(4):e95266 Sim C S F, Cheow Y L, Ng S L, et al. Discovering metal-tolerant endophytic fungi from the phytoremediator plant Phragmites[J]. Water, Air, & Soil Pollution, 2018, 229(3):1-11 Zhou Y, Li X, Gao Y, et al. Plant endophytes and arbuscular mycorrhizal fungi alter plant competition[J]. Functional Ecology, 2018, 32(5):1168-1179 Field K J, Rimington W R, Bidartondo M I, et al. Functional analysis of liverworts in dual symbiosis with Glomeromycota and Mucoromycotina fungi under a simulated Palaeozoic CO2 decline[J]. The ISME Journal, 2016, 10(6):1514-1526 Shanmugaiah V, Balasubramanian N, Gomathinayagam S, et al. Effect of single application of Trichoderma viride and Pseudomonas fluorescens on growth promotion in cotton plants[J]. African Journal of Agricultural Research, 2009, 4(11):1220-1225 Hiruma K, Gerlach N, Sacristán S, et al. Root endophyte Colletotrichum tofieldiae confers plant fitness benefits that are phosphate status dependent[J]. Cell, 2016, 165(2):464-474 Agler M T, Ruhe J, Kroll S, et al. Microbial hub taxa link host and abiotic factors to plant microbiome variation[J]. PLoS Biology, 2016, 14(1):e1002352 Purahong W, Hyde K D. Effects of fungal endophytes on grass and non-grass litter decomposition rates[J]. Fungal Diversity, 2011, 47(1):1-7 White J F, Kingsley K L, Verma S K, et al. Rhizophagy cycle:An oxidative process in plants for nutrient extraction from symbiotic microbes[J]. Microorganisms, 2018, 6(3):E95 Gadd G M. Fungal production of citric and oxalic acid:Importance in metal speciation, physiology and biogeochemical processes[J]. Advances in Microbial Physiology, 1999, 41:47-92 Devi L S, Joshi S R. Ultrastructures of silver nanoparticles biosynthesized using endophytic fungi[J]. Journal of Microscopy and Ultrastructure, 2015, 3(1):29-37 Singh P, Kim Y J, Zhang D B, et al. Biological synthesis of nanoparticles from plants and microorganisms[J]. Trends in Biotechnology, 2016, 34(7):588-599 DalCorso G, Manara A, Furini A. An overview of heavy metal challenge in plants:From roots to shoots[J]. Metallomics:Integrated Biometal Science, 2013, 5(9):1117-1132 Zahoor M, Irshad M, Rahman H, et al. Alleviation of heavy metal toxicity and phytostimulation of Brassica campestris L. by endophytic Mucor sp. MHR-7[J]. Ecotoxicology and Environmental Safety, 2017, 142:139-149 Na G, Salt D E. The role of sulfur assimilation and sulfur-containing compounds in trace element homeostasis in plants[J]. Environmental and Experimental Botany, 2011, 72(1):18-25 Freeman J L, Persans M W, Ken N M, et al. Increased glutathione biosynthesis plays a role in nickel tolerance in Thlaspi nickel hyperaccumulators[J]. The Plant Cell, 2004, 16(8):2176-2191 Zhao D K, Li T, Shen M, et al. Diverse strategies conferring extreme cadmium (Cd) tolerance in the dark septate endophyte (DSE), Exophiala pisciphila:Evidence from RNA-seq data[J]. Microbiological Research, 2015, 170:27-35 Adriaensen K, van der Lelie D, van Laere A, et al. A zinc-adapted fungus protects pines from zinc stress[J]. New Phytologist, 2004, 161(2):549-555 Tang Y Z, Shi L, Zhong K C, et al. Ectomycorrhizal fungi may not act as a barrier inhibiting host plant absorption of heavy metals[J]. Chemosphere, 2019, 215:115-123 Hartley J, Cairney J W G, Meharg A A. Do ectomycorrhizal fungi exhibit adaptive tolerance to potentially toxic metals in the environment?[J]. Plant and Soil, 1997, 189(2):303-319 Lastovetsky O A, Gaspar M L, Mondo S J, et al. Lipid metabolic changes in an early divergent fungus govern the establishment of a mutualistic symbiosis with endobacteria[J]. Proceedings of the National Academy of Sciences of the United States of America, 2016, 113(52):15102-15107 Uroz S, Calvaruso C, Turpault M P, et al. Effect of the mycorrhizosphere on the genotypic and metabolic diversity of the bacterial communities involved in mineral weathering in a forest soil[J]. Applied and Environmental Microbiology, 2007, 73(9):3019-3027 Fransson P, Andersson A, Norström S, et al. Ectomycorrhizal exudates and pre-exposure to elevated CO2 affects soil bacterial growth and community structure[J]. Fungal Ecology, 2016, 20:211-224 Krupa P, Kozdrój J. Ectomycorrhizal fungi and associated bacteria provide protection against heavy metals in inoculated pine (Pinus sylvestris L.) seedlings[J]. Water, Air, and Soil Pollution, 2007, 182(1-4):83-90 Strandberg G W, Shumate S E, Parrott J R. Microbial cells as biosorbents for heavy metals:Accumulation of uranium by Saccharomyces cerevisiae and Pseudomonas aeruginosa[J]. Applied and Environmental Microbiology, 1981, 41(1):237-245 Gadd G M. Interactions of Fungi with Toxic Metals[M]//The Genus Aspergillus. Boston:Springer US, 1994:361-374 Fogarty R V, Tobin J M. Fungal melanins and their interactions with metals[J]. Enzyme and Microbial Technology, 1996, 19(4):311-317 Bhanoori M, Venkateswerlu G. In vivo chitin-cadmium complexation in cell wall of Neurospora crassa[J]. Biochimica et Biophysica Acta, 2000, 1523(1):21-28 Martino E, Coisson J D, Lacourt I, et al. Influence of heavy metals on production and activity of pectinolytic enzymes in ericoid mycorrhizal fungi[J]. Mycological Research, 2000, 104(7):825-833 Ashford A E, Allaway C A, Peterson C A, et al. Nutrient transfer and the fungus-root interface[J]. Functional Plant Biology, 1989, 16(1):85 Fomina M A, Alexander I J, Colpaert J V, et al. Solubilization of toxic metal minerals and metal tolerance of mycorrhizal fungi[J]. Soil Biology and Biochemistry, 2005, 37(5):851-866 Meharg A A. The mechanistic basis of interactions between mycorrhizal associations and toxic metal cations[J]. Mycological Research, 2003, 107(11):1253-1265 Colpaert J V, Assche J A. The effects of cadmium on ectomycorrhizal Pinus sylvestris L.[J]. New Phytologist, 1993, 123(2):325-333 Jones M D, Hutchinson T C. The effect of mycorrhizal infection on the response of Betula papyrifera to nickel and copper[J]. New Phytologist, 1986, 102(3):429-442 Denny H J, Wilkins D A. Zinc tolerance in Betula spp. Ⅳ. The mechanism of ectomycorrhizal amelioration of zinc toxicity[J]. New Phytologist, 1987, 106(3):545-553 Ashford A E, Peterson R L, Dwarte D, et al. Polyphosphate granules in eucalypt mycorrhizas:Determination by energy dispersive X-ray microanalysis[J]. Canadian Journal of Botany, 1986, 64(3):677-687 Zarb J, Walters D R. Polyamine biosynthesis in the ectomycorrhizal fungus Paxillus involutus exposed to lead[J]. Mycological Research, 1996, 100(4):486-488 Bellion M, Courbot M, Jacob C, et al. Extracellular and cellular mechanisms sustaining metal tolerance in ectomycorrhizal fungi[J]. FEMS Microbiology Letters, 2006, 254(2):173-181 Trotter E W, Grant C M. Thioredoxins are required for protection against a reductive stress in the yeast Saccharomyces cerevisiae[J]. Molecular Microbiology, 2002, 46(3):869-878 Ott T, Fritz E, Polle A, et al. Characterisation of antioxidative systems in the ectomycorrhiza-building basidiomycete Paxillus involutus (Bartsch) Fr. and its reaction to cadmium[J]. FEMS Microbiology Ecology, 2002, 42(3):359-366 Kalsotra T, Khullar S, Agnihotri R, et al. Metal induction of two metallothionein genes in the ectomycorrhizal fungus Suillus himalayensis and their role in metal tolerance[J]. Microbiology, 2018, 164(6):868-876 Colpaert J V, Wevers J H L, Krznaric E, et al. How metal-tolerant ecotypes of ectomycorrhizal fungi protect plants from heavy metal pollution[J]. Annals of Forest Science, 2011, 68(1):17-24 Wężowicz K, Rozpądek P, Turnau K. The diversity of endophytic fungi in Verbascum lychnitis from industrial areas[J]. Symbiosis, 2014, 64(3):139-147 -
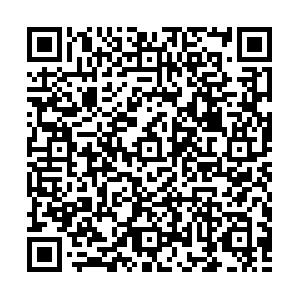
计量
- 文章访问数: 2507
- HTML全文浏览数: 2507
- PDF下载数: 95
- 施引文献: 0