基于T-Hg2+-T结构的Hg2+生物传感器研究进展
Research progress of Hg2+ biosensor based on T-Hg2+-T structure
-
摘要: Hg2+不仅污染环境,还会引发多种疾病,危害人体健康.因此,实现对Hg2+的快速灵敏检测十分必要.传统的Hg2+检测方法存在一定的局限性,而基于T-Hg2+-T结构的汞离子生物传感器具有高特异性、高稳定性的优势,近些年得到广泛研究和应用.本文简要介绍了T-Hg2+-T结构的概念和特点,并从荧光型、比色型和电化学型3个方面综述了基于T-Hg2+-T结构的汞离子生物传感器的研究进展,对未来的发展前景进行了展望.Abstract: Hg2+ not only results in environmental pollution, but also causes a variety of diseases and is harmful to human health. Therefore, it is essential to achieve the rapid and sensitive detection of Hg2+. Traditional Hg2+ detection methods have some limitations in applications. Recently, Hg2+ biosensors based on T-Hg2+-T structure have been widely studied and applied because of their high specificity and stability. This paper briefly introduced the concept and characteristics of T-Hg2+-T structure and reviewed the research progress of fluorescent, colorimetric and electrochemical Hg2+ biosensors based on T-Hg2+-T structure. The future prospect of such kind of biosensor was also discussed.
-
Key words:
- biosensor /
- mercury ion /
- T-Hg2+-T /
- fluorescent assay /
- electrochemical assay
-
“双碳”背景下,绿色低碳的污水处理技术成为发展重点。好氧颗粒污泥 (aerobic granular sludge,AGS) 是微生物自凝聚形成的颗粒状活性污泥,具有沉降性能好、生物量高、可同步去除碳氮磷等优点,而相比于传统活性污泥工艺,AGS能节省50%~75%的占地面积、20%~25%的运行费用和23%~40%的电耗,故该技术符合当前减污降碳的发展目标,具有一定应用前景[1]。世界范围内已有80余座污水处理厂在序批式反应器 (sequencing batch reactor,SBR) 中成功应用了AGS技术,但在连续流反应器中成功应用AGS技术的仅10余座,连续流AGS的推广应用还未取得实质性的突破[2]。尽管SBR更易于培养AGS,但存在处理量小、设备使用率低等缺陷。而连续流是现有污水处理厂的主要运行模式,故连续流AGS的培养备受关注。
丰盛-饥饿条件[3-4]、基于污泥沉降速度[5-6]、尺寸或密度[7-8]的选择压及水力剪切力[9]等被认为是SBR-AGS颗粒化的关键影响因素。但连续流的培养环境与SBR截然不同,这些关键影响因素更难实现。SUN等[10]采用系列串联的完全混合反应器组成整体推流的连续流系统,同时沉淀池采用1 min进水→4 min静态沉淀→1 min排水的间歇运行模式,创造基于沉降速度的选择压,研究了丰盛-饥饿条件对连续流AGS形成的必要性。LIU等[11-12]应用双区沉淀池,通过调整沉淀区上方挡板的高度设置污泥选择压,在AAO系统中培养出平均粒径为210 µm的AGS。以上研究证实了丰盛-饥饿条件和选择压在连续流AGS培养中的必要性和可行性,但沉淀池的运行策略仍较复杂。在厌氧颗粒污泥的研究中,顶部为三相分离器的升流式反应器[13-14]能很好地富集颗粒污泥,但这些研究大多采用大高径比的柱式反应器,与现有污水处理厂的平铺式构筑物不兼容,开发的培养策略难以直接应用。再加上现有研究多为接种成熟AGS的小试实验,缺乏直接在连续流模式下培养AGS的更大尺度研究。
基于此,本团队提出一种新型的连续流AGS反应器,将三相分离器与传统活性污泥工艺组合,构成反应耦合沉淀一体式的反应器,以中试尺度在现有污水处理厂进行改造应用。通过向系统中接种活性污泥,以低浓度市政污水为基质,探究AGS的形成过程及其形貌和结构特性,并通过监测中试系统对NH4+-N和TN的去除效果,再结合微生物群落结构角度以探索系统的脱氮机理,以期为连续流AGS这一绿色低碳处理工艺的应用推广提供参考。
1. 材料与方法
1.1 中试系统及运行方法
中试系统位于河北省某市政污水处理厂原厌氧池,日处理量为3 000 m3。该厂出水标准由《城镇污水处理厂污染物排放标准》 (GB18918-2002) 一级A改为执行地方标准,其中COD、NH4+-N和TP的排放浓度限值分别由50、5 (8) 和0.5 mg·L−1降为40、2.0 (3.5) 和0.4 mg·L−1,SS、BOD5和TN不变。根据该厂2019年的运行数据,提标后现有工艺出水NH4+-N和TN存在超标风险。
中试系统由原厌氧池改造而来 (图1) ,该系统分为I和II两个系列,均由微氧池、好氧池及置于好氧池内部、基于三相分离器的沉淀分离装置组成。系列I:微氧池11.0 m×6.0 m×6.5 m,好氧池13.5 m×6.0 m×3.8 m,好氧池内沉淀分离装置13.5 m×6.0 m×1.0 m;系列II:微氧池13.8.0 m×6.0 m×6.5 m,好氧池13.5 m×6.0 m×3.8 m,好氧池内沉淀分离装置13.5 m×6.0 m×1.0 m。进水流量、污泥回流量、剩余污泥外排量及曝气量均采用变频控制器控制。污泥质量浓度保持在4~7 g·L−1,采用气提回流控制污泥回流比约200%,每日排泥控制污泥龄26~30 d,调整曝气量使得微氧池溶解氧 (DO) 为0.2~0.5 mg·L−1,好氧池DO为1.0~3.0 mg·L−1。与传统活性污泥工艺相比,中试系统有如下特点:1) 未设缺氧池,前置的反应器为微氧池,旨在通过控制微量曝气以充分利用原水中的碳源实现同步硝化反硝化脱氮;2) 通过好氧池内置的沉淀分离装置完成固-液-气三相分离,省去二沉池,减少占地面积,同时内、外回流合二为一,可降低运行能耗。
1.2 原水水质及接种污泥
中试系统进水为该污水处理厂旋流沉砂池出水,其水质指标如下:COD (236.5±51.6) mg·L−1,NH4+-N (57.3±14.6) mg·L−1,TN (67.2±14.7) mg·L−1,TP (6.0±1.7) mg·L−1。原水C/N仅为3.6,生物脱氮碳源不足,故在微氧池定量投加乙酸钠作为外加碳源。这使得实际进水COD为 (328.1±73.6) mg·L−1,C/N为4.9。
中试系统接种该厂生化池活性污泥,其混合液悬浮固体浓度 (MLSS) 为4.2 g·L−1,挥发性悬浮固体浓度 (MLVSS) 为1.9 g·L−1,污泥指数 (SVI30) 为59.4 mL·g−1,粒径分布 (D50) 为28.9 μm,是典型的絮状活性污泥。
1.3 分析与检测方法
中试系统于2021年1月24日完成改造和接种工作,进出水采样时间为4月21日至7月5日。这段时间中试系统处于稳定运行状态,出水水质足以评估中试系统的污染物去除效果。采用纳氏分光光度法测定进出水NH4+-N;采用碱性过硫酸钾消解紫外分光光度法测定TN;DO和pH采用便携式测定仪 (HACH,HQ30d,美国) 测定。
采用激光粒度仪 (Beckman,LS13320,美国) 测定接种污泥的粒径分布,并于7月13日和9月13日在中试系统中进行采样。采用数码相机观察AGS的宏观形态,通过扫描电子显微镜 (SEM) 观察AGS的微观结构及微生物形态。SEM样品制备过程[15]:用4%戊二醛固定;再用去离子水多次浸泡冲洗;经50%、70%、85%、95%、100%乙醇梯度脱水;临界点干燥、粘样、镀膜观察。通过流变仪 (Aaton Paar Physica MCR301,奥地利) 测定动态粘弹性。并分析了中试AGS与接种污泥的流变特性差异。具体步骤如下:采用平板-平板测量系统,平板直径50 mm,用一次性滴管滴加样品,刮除平板外多余样品后,在固定角频率5 rad·s−1、温度25 ℃条件下测定[16]。此外,为探究AGS中微生物群落结构的变化,使用孔径0.2 mm的筛网收集中试中粒径<0.2 mm (命名为F) 和>0.2 mm (命名为G) 及接种污泥样品 (命名为AS) 委托上海美吉生物医药科技有限公司进行16S rRNA高通量测序及种群分析。
2. 结果与讨论
2.1 污泥形态与粒径变化
图2 (a) 表明,在宏观上中试系统好氧池中的原始污泥与接种污泥几乎没有差别,但中试系统中的污泥经孔径为0.2 mm的筛网筛分后可得到粒径较大的AGS,采用数码相机和SEM观察AGS的形貌,结果如图2 (b)~(d) 所示。中试系统筛分得到的AGS轮廓清晰、紧致饱满,为形状规则的球形和椭球形。SEM图像表明AGS微观上主要由球状菌紧密相连,表面有少量丝状菌,这与文献[17]报道的实验室培养AGS形貌非常相似。这表明中试系统在连续流模式下,接种絮状活性污泥后使用实际低浓度低C/N的市政污水已成功培养出了AGS。
图3为接种污泥及中试系统稳定运行过程中污泥粒径分布的变化情况。粒径分布曲线右移表明随着中试系统的运行,污泥粒径逐渐增大。接种污泥的D50为28.9 μm。到了7月13日,中试系统中污泥D50为57.8 μm;到了9月13日,D50进一步增大到90.1 μm,较接种污泥粒径增大了3.1倍。粒径区间分布发现,接种污泥粒径主要分布在<50 μm,其占比高达76.8%,少量污泥粒径分布在50~100 μm,占比17.1%。但到7月13日,中试系统中污泥粒径较均匀地分布到了<50 μm、50~100 μm及100~150 μm,占比分别为42.2%、31.7%及20.3%。粒径为150~200 μm的污泥占比由初始的1.0%增至4.9%。到了9月13日,更是增加到10.3%。而粒径>200 μm的污泥占比由初始的0.9%增至9.4%,实现了数量级跃增。连续流模式下培养的AGS粒径小于SBR中培养的AGS粒径[18],这是由于连续流系统往往处于完全混合的状态,反应器内的基质浓度与出水浓度相当,更低的基质浓度意味着更小的扩散内径,从而限制了连续流中AGS的粒径。此外,本研究中污泥的颗粒化比例 (粒径>200 μm的污泥占比) 低于文献[12, 19]的报道值。这是由于中试系统中没有设置污泥选择压,系统中形成的AGS与絮状污泥一起作为剩余污泥被排出,不利于AGS的富集,从而导致颗粒化比例较低。
2.2 污泥流变特性
接种污泥及中试系统培养的AGS的流变特性如图4所示。通过动态应变扫描确定污泥的线性黏弹区,接种污泥和AGS的临界应变点均在1%左右。当应变超过线性黏弹区后,储能模量G´开始下降,这表明污泥样品的结构开始被破坏。2份污泥样品的G´均大于G´´,说明污泥样品具有凝胶状或固体状的结构,可被称为黏弹性固体。此外,由实验数据可得到污泥样品的屈服应力τy,即黏弹性极限处的剪切应力和流动应力τf,即样品变形过程G´=G´´处的剪切应力。接种污泥和AGS的τy分别为2.2和37.3 Pa,τf分别为7.9和390.3 Pa。这说明中试系统培养的AGS机械强度远高于接种的活性污泥。这主要是由于AGS中胞外多聚物 (extracellular polymeric substance,EPS) 的含量往往高于活性污泥,EPS将AGS中的微生物团聚在一起,从而使得AGS比絮状污泥有更高的机械强度。
2.3 污染物去除效果
中试系统处理水量情况如图5 (a) 所示。除6月3日、6月14日和15日由于进水泵故障,其余时间中试系统处理量均略高于设计值,I、II系列平均日处理量分别为1 454.9和1 722.4 m3·d−1。由图5 (b) 可知,原水COD呈现出明显的雨季降低的变化趋势,在进入雨季前 (4—5月) ,原水COD稳定在 (267.7±30.2) mg·L−1,而进入雨季 (6—7月) 后,平均COD降至 (200.1±54.9) mg·L−1。这是由于尽管该厂收水区域的排水体制为分流制,但可能存在管网的错接、混接等问题,导致雨季部分雨水进入污水管网,从而使得污水处理厂进水COD偏低。原水C/N为 (3.6±0.6) ,属于典型的低C/N污水,生物脱氮难度大。因此,需要定量投加乙酸钠作为碳源,而保证进水COD达到 (328.1±73.6) mg·L−1,C/N达 (4.9±0.6) 。
中试系统对NH4+-N和TN的去除情况如图5 (c) 和 (d) 所示。与进水COD类似,进水NH4+-N和TN在雨季呈现出明显降低的趋势。在进入雨季前,进水NH4+-N和TN分别为 (68.5±4.5) mg·L−1和 (78.2±4.5) mg·L−1;在进入雨季后,分别降为 (45.9±14.7) mg·L−1和 (55.6±15.7) mg·L−1。I系列和II系列出水NH4+-N分别为 (1.3±1.1) mg·L−1和 (1.0±0.8) mg·L−1,平均去除率分别为97.7%和98.2%。出水NH4+-N基本保持在2 mg·L−1以下,满足地方标准要求的2.0 mg·L−1,达标时间占比分别为81.8%和93.5%。其中,I系列由于曝气设备故障导致超标时间多于II系列。出水TN分别为 (9.9±2.8) mg·L−1和 (9.1±2.6) mg·L−1,平均去除率分别为84.8%和85.7%。出水TN基本保持在10 mg·L−1左右,满足地方标准要求的15.0 mg·L−1,达标时间占比分别为94.8%和96.1%。此外,中试系统不仅获得了良好的出水水质,而且由于二沉池耦合在好氧池内部,省去了二沉池,可减小占地面积,同时硝化液回流和污泥回流合二为一,能降低系统的运行能耗。
2.4 微生物群落结构分析
为探究中试系统中污泥微生物群落结构的变化,使用孔径0.2 mm的筛网收集粒径<0.2 mm (F) 和>0.2 mm (G) 及接种污泥样品 (AS) 进行高通量测序。如表1所示,Coverage指数均大于99%,表明测序的结果具有代表性。Alpha多样性分析中的Simpson和Shannon指数均用于反映群落多样性;Simpson指数越大说明群落多样性越低;而Shannon值越大说明群落多样性越高。中试系统中F和G的Simpson指数均大于AS的,而Shannon值则均小于AS的。这表明中试系统污泥群落多样性减少,这可能与中试系统条件下能选择性富集功能微生物有关。
表 1 样本Alpha多样性指数Table 1. Alpha-diversity of the samples样本 Simpson Shannon Coverage AS 0.013 5.5 99.3% F 0.059 4.7 99.2% G 0.060 4.9 99.3% 微生物群落结构组成分析如图6所示。在门水平上,AS、F和G中优势菌群类似,均为Proteobacteria (34.45%、46.53%和50.51%),Chloroflexi (17.36%,19.74%和16.58%) 和Bacteroidota (15.03%,9.92%和13.00%) 。这3类微生物在营养物的去除中具有重要作用[20],是污水处理中常见的微生物。除这3类优势菌群外,其余菌群丰度也发生了较大变化,如Actinobacteriota在AS中的相对丰度为13.08%,但在F中为6.37%,在G仅为4.39%;类似的还有Patescibacteria,其在AS中的相对丰度为5.27%,但在F和G的相对丰度仅为1.56%和1.19%;而Firmicutes在AS中的相对丰度为3.11%,尽管在F中增加到了6.26%,但在G中仅为2.30%。这表明相比于接种污泥,中试系统中的污泥微生物群落结构发生了变化,而且粒径<0.2 mm的污泥与粒径>0.2 mm的污泥微生物群落结构也有显著的差异。在属水平上,接种污泥中的优势属为Methylophilaceae (6.10%) ,Microtrichales (5.28%) ,Methylotenera (4.81%) ,Saprospiraceae (4.28%) 和Saccharimonadales (4.30%) 。而在中试系统中,Methylophilaceae和Methylotenera得到了显著富集,F和G中的丰度分别为23.73%和6.34%,24.61%和7.16%,丰度和为AS的3倍以上。Methylophilaceae和Methylotenera普遍存在于自然环境中,包括淡水、土壤、污水等生态位,是一种兼性厌氧、以甲醇等为生长基质的甲基营养型细菌,因其能在有氧条件下进行反硝化而受到关注[21]。中试系统由微氧池和好氧池组成,而没有设置缺氧池,NH4+-N在微氧池或好氧池中被氧化,同时生成的NO2−-N/NO3−-N的也只能在微氧池或好氧池被还原成N2,这可能是中试系统大量富集好氧反硝化菌Methylophilaceae和Methylotenera的原因。同时,异养菌Microtrichales和Saccharimonadales的丰度则显著下降,由AS中的5.28%和4.30%分别降低至1.80%和1.11%,1.09%和0.57%,这可能与系统中有机物利用的途径有关。
2.5 颗粒化及脱氮机理分析
新型微氧-好氧耦合沉淀一体式反应器构型对AGS的形成、系统脱氮性能及微生物群落结构变化有着重要影响。在好氧池内,底部曝气和微氧池出水 (即好氧池进水) 产生推动力,使得好氧池内部混合液向上流动,与内置的三相分离器碰撞并实现固液气三相分离。分离的气体经三相分离器间的气-液平面逸散到空气中,液体经出水渠排出系统,而泥水混合液只能向下继续流动,在好氧区与三相分离器间形成剧烈的内循环流动,为AGS的形成提供关键的驱动力-水力剪切力。水力剪切力在颗粒化初期能促进微生物的随机运动,增加微生物间的有效碰撞,有利于形成初始可逆的微生物聚集体[22]。诱导EPS的分泌可增强细胞表面的疏水性,增加聚集体的密度,从而进一步形成不可逆的微生物聚集体[23]。在稳定阶段,水力剪切力不断剥离成熟AGS表面附着生长的丝状菌,维持AGS的形貌和优势地位[5]。这与DAI 等[24]的发现类似,即由反应器内部纵向循环产生水力剪切力培养AGS的机理。此外,沉淀耦合在好氧池内部,省去了二沉池,可节省占地面积,同时将内、外回流合二为一,降低运行能耗。
在微氧池中,DO控制在0.2~0.5 mg L−1,当微氧池COD为50~100 mg L−1时,DO可渗透AGS外表层10~18 µm处[25],故粒径大于20~36 µm的AGS内可形成外层好氧、内层缺氧/厌氧的分层结构。进水中的NH4+-N在外层好氧条件下被氧化成NOx−-N,生成的NOx−-N扩散至内层,在内层缺氧/厌氧的条件下发生反硝化,使得系统可在微氧池中完成生物脱氮。但这一脱氮途径只存在于AGS形成后。在启动初期,接种的絮状污泥无法通过该途径完成生物脱氮,系统脱氮性能较差;在启动中期,随着中试系统的运行,尽管AGS还未形成,但系统富集了大量的好氧反硝化菌Methylophilaceae和Methylotenera,能通过好氧反硝化途径完成脱氮;而在AGS形成后的稳定运行阶段,两种脱氮途径共同完成生物脱氮过程。此外,微氧池中的反硝化过程不仅能充分利用原水中的碳源,还能降低进入好氧池中的有机物浓度,创造微氧池丰盛-好氧池饥饿的运行条件,有利于AGS的长期稳定性。
3. 结论
1) 以低浓度市政污水为基质、接种活性污泥,在中试规模 (3 000 m3 d−1) 的连续流运行模式下,可培养出长期稳定存在的AGS,平均粒径由接种污泥的28.9 μm增至90.1 μm,其中粒径>100 μm的占47.8%,>200 μm的占9.4%。
2) 中试系统I、II系列出水NH4+-N分别为 (1.3±1.1) mg·L−1和 (1.0±0.8) mg·L−1,出水TN分别为 (9.9±2.8) mg·L−1和 (9.1±2.6) mg·L−1,系统具有良好的脱氮效果,能满足该厂的提标改造要求。
3) 中试系统大量富集了好氧反硝化菌Methylophilaceae和Methylotenera,好氧反硝化途径可能在脱氮中起重要作用。微氧池中的反硝化过程能充分利用原水碳源,降低进水有机物浓度,有利于维持系统的稳定高效低碳运行。
-
[1] KUMARI S, AMIT, JAMWAL R, et al. Recent developments in environmental mercury bioremediation and its toxicity:A review[J]. Environmental Nanotechnology, Monitoring & Management, 2020, 13:100238. [2] NATASHA, SHAHID M, KHALID S, et al. A critical review of mercury speciation, bioavailability, toxicity and detoxification in soil-plant environment:Ecotoxicology and health risk assessment[J]. The Science of the Total Environment, 2020, 711:134749. [3] JEEVANANTHAM S, SARAVANAN A, HEMAVATHY R V, et al. Removal of toxic pollutants from water environment by phytoremediation:A survey on application and future prospects[J]. Environmental Technology & Innovation, 2019, 13:264-276. [4] LI Q, ZHANG Z, WANG Z. Determination of Hg2+ by on-line separation and pre-concentration with atmospheric-pressure solution-cathode glow discharge atomic emission spectrometry[J]. Analytica Chimica Acta, 2014, 845:7-14. [5] 胡敬芳, 李玥琪, 高国伟, 等. 水质重金属检测技术研究进展[J]. 传感器世界, 2017, 23(7):7-15. HU J F, LI Y Q, GAO G W, et al. Research progress in heavy metal detection technologies in water[J]. Sensor World, 2017, 23(7):7-15(in Chinese).
[6] LUOH, WANG X, DAI R, et al. Simultaneous determination of arsenic and cadmium by hydride generation atomic fluorescence spectrometry using magnetic zero-valent iron nanoparticles for separation and pre-concentration[J]. Microchemical Journal, 2017, 133:518-523. [7] KHANSILI N, RATTU G, KRISHNA P M, et al. Label-free optical biosensors for food and biological sensor applications[J]. Sensors and Actuators B:Chemical, 2018, 265:35-49. [8] 黄鹏宇, 李奕君, 张天牧, 等.水中磺胺对甲氧嘧啶抗生素的平面波导免疫传感器检测[J]. 环境化学,2020, 39(2):433-440. HUANG P Y, LI Y J, ZHANG T M, et al. Detection of sulfameter in water by a planar waveguide immunosensor[J]. Environmental Chemistry, 2020, 39(2):433-440(in Chinese).
[9] 李伟, 吴君, 王浩宇, 等.倏逝波免疫传感器超灵敏检测水样中的双酚A[J].环境化学,2018,37(2):347-352. LI W, WU J, WANG H Y, et al. Ultrasensitive detection of BPA in water using an evanescent wave immunosensor[J]. Environmental Chemistry, 2018, 37(2):347-352(in Chinese).
[10] MADURAIWEERAN G, SASIDHARAN M, GANESAN V. Electrochemical sensor and biosensor platforms based on advanced nanomaterials for biological and biomedical applications[J]. Biosensors and Bioelectronics, 2018, 103:113-129. [11] 王蓉, 孔丹丹, 杨世海, 等.电化学生物传感器技术在重金属快速检测领域中的研究进展[J]. 分析试验室, 2019, 38(11):1366-1373. WANG R, KONG D D, YANG S H, et al. Recent advances in various electrochemical biosensor for heavy metal[J]. Chinese Journal of Analysis Laboratory, 2019, 38(11):1366-1373(in Chinese).
[12] 杜再慧, 李相阳, 田晶晶, 等. 汞离子功能核酸生物传感器的建立与应用[J]. 分析化学, 2018, 46(6):947-951 DU Z H, LI X Y, TIAN J J, et al. Establishment and application of functional nucleic acid biosensor for detection of mercury ion[J].Chinese Journal of Analytical Chemistry, 2018, 46(6):947-951(in Chinese).
[13] TORRIGORe H, ONO A, KOZASA T, et al. HgⅡ ion specifically binds with T:T mismatched base pair in duplex DNA[J]. Chemistry A European Journal, 2010, 16(44):13218-13225. [14] YOKO M, HUMIKA T, MITSURU T, et al. MercuryⅡ-mediated formation of thymine-HgⅡ-thymine base pairs in DNA duplexes[J]. Journal of the American Chemical Society, 2006, 128:2172-2173. [15] TANAKA Y, ODA S, YAMAGUCHI H, et al. 15N-15N J-coupling across Hg(Ⅱ):direct observation of Hg(Ⅱ)-mediated T-T base pairs in a DNA duplex[J]. Journal of the American Chemical Society, 2007, 129(2):244-245. [16] TORIGOE H, MIYAKAWA Y, ONO A, et al. Positive cooperativity of the specific binding between Hg2+ ion and T:T mismatched base pairs in duplex DNA[J]. Thermochimica Acta, 2012, 532:28-35. [17] ONO A, TORIGOE H, TANAKA Y, et al. Binding of metal ions by pyrimidine base pairs in DNA duplexes[J]. Chemical Society Reviews, 2011, 40(12):5855-5866. [18] HIROSHI Y, ŠEBERA J, KONDO J, et al. The structure of metallo-DNA with consecutive thymine-HgⅡ-thymine base pairs explains positive entropy for the metallo base pair formation[J]. Nucleic acids research, 2013, 42(6):4094-4099. [19] 马佳, 刘楠, 马新华, 等. 双链DNA中汞/银离子-嘧啶碱基对间结合作用及其在检测技术方面的应用研究进展[J]. 应用与环境生物学报, 2015, 21(5):848-853. MA J, LIU N, MA X H, et al. Review on the study of mercury/silver ions-pyrimidine base pairs in DNA duplexes and their application in detection technology[J]. Chinese Journal of Applied and Environmental Biology, 2015, 21(5):848-853(in Chinese).
[20] 张何, 赵智粮, 傅昕, 等. 基于分子间裂分G-四链体-氯化血红素DNA酶自组装纳米线的"Turn-on"型汞离子传感研究[J]. 传感技术学报, 2018, 31(12):1822-1827. ZHANG H, ZHAO Z L, FU X, et al. "Turn-on" sensor for detection of mercury ion through autonomous assembly of intermolecular splitting G-quardruplex-hemin DNazyme nanowires[J]. Chinese Journal of Sensors and Actuators, 2018, 31(12):1822-1827(in Chinese).
[21] KHOSHBIN Z, HOUSAINDOKHT M R, VERDIAN A, et al. Simultaneous detection and determination of mercury (Ⅱ) and lead (Ⅱ) ions through the achievement of novel functional nucleic acid-based biosensors[J]. Biosensors and Bioelectronics, 2018, 116:130-147. [22] LI H H, HUANG X Q, HASSAN M Md, et al. Dual-channel biosensor for Hg2+ sensing in food using Au@Ag/graphene-upconversion nanohybrids as metal-enhanced fluorescence and SERS indicators[J]. Microchemical Journal, 2020, 154:104563. [23] LIU Y Y, CAI Z X, SHENG L, et al. A magnetic relaxation switching and visual dual-mode sensor for selective detection of Hg2+ based on aptamers modified Au@Fe3O4 nanoparticles[J]. Journal of Hazardous Materials, 2020, 388:121728. [24] ONO A, TOGASHI H. Highly Selective oligonucleotide-based sensor for Mercury(Ⅱ) in aqueous solutions[J]. Angewandte Chemie (International ed. in English), 2004, 43(33):4300-4302. [25] WANG R, ZHOU X, SHI H, et al. T-T mismatch-driven biosensor using triple functional DNA-protein conjugates for facile detection of Hg2+[J]. Biosensors and Bioelectronics, 2016, 78:418-422. [26] HAN S T, ZHOU X H, TANG Y F, et al. Practical, highly sensitive, and regenerable evanescent-wave biosensor for detection of Hg2+ and Pb2+ in water[J]. Biosensors and Bioelectronics, 2016, 80:265-272. [27] CUI X, ZHU L, WU J, et al. A fluorescent biosensor based on carbon dots-labeled oligodeoxyribonucleotide and graphene oxide for mercury (Ⅱ) detection[J]. Biosensors and Bioelectronics, 2015, 63:506-512. [28] YUN W, XIONG W, WU H, et al. Graphene oxide-based fluorescent "turn-on" strategy for Hg2+ detection by using catalytic hairpin assembly for amplification[J]. Sensors and Actuators B:Chemical, 2017, 249:493-498. [29] ZUO X, ZHANG H, ZHU Q, et al. A dual-color fluorescent biosensing platform based on WS2 nanosheet for detection of Hg2+ and Ag+[J]. Biosensors and Bioelectronics, 2016, 85:464-470. [30] 左显维,冯治棋,胡艳琴,等.基于MoS2纳米片的荧光生物传感器对饮用水中Hg2+的检测[J].环境化学,2017,36(1):167-174. ZUO X W, FENG Z Q, HU Y Q, et al. A fluorescent biosensor based on MoS2 nanosheets for the detection of Hg2+ in drinking water[J]. Environmental Chemistry, 2017, 36(1):167-174.
[31] RAVIKUMAR A, PANNEERSELVAM P. Polydopamine nanotube mediated fluorescent biosensor for Hg(Ⅱ) determination through exonuclease Ⅲ-assisted signal amplification[J]. Analyst, 2018, 143(11):2623-2631. [32] GUO H, LI J S, LI Y W, et al. Exciton energy transfer-based fluorescent sensor for the detection of Hg2+ through aptamer-programmed self-assembly of QDs[J]. Analytica Chimica Acta, 2019, 1048:161-167. [33] SHAN Y, WANG B, HUANG H, et al. On-site quantitative Hg2+ measurements based on selective and sensitive fluorescence biosensor and miniaturized smartphone fluorescence microscope[J]. Biosensors & Bioelectronics, 2019, 132:238-247. [34] LI Y, LIU N, LIU H, et al. A novel label-free fluorescence assay for one-step sensitive detection of Hg2+ in environmental drinking water samples[J]. Scientific Reports, 2017, 7:45974. [35] 朱锡宇, 王若瑜, 周小红, 等. 基于功能核酸的水中汞离子荧光检测方法[J]. 光谱学与光谱分析, 2018, 38(11):3447-3451. ZHU X Y, WANG R Y, ZHOU X H, et al. Functional nucleic acid based fluorescent biosensing method for Hg2+ detection in water samples[J]. Spectroscopy and Spectral Analysis, 2018, 38(11):3447-3451(in Chinese).
[36] SONG X L, FU B C, LAN Y F, et al. Label-free fluorescent aptasensor berberine-based strategy for ultrasensitive detection of Hg2+ ion[J]. Spectrochimica Acta Part A:Molecular and Biomolecular Spectroscopy, 2018, 204:301-307. [37] LI Z H, SUN H J, MA X Y, et al. Label-free fluorescence "turn-on" strategy for mercury (Ⅱ) detection based on the T-Hg2+-T configuration and the DNA-sensitized luminescence of terbium(Ⅲ)[J]. Analytica Chimica Acta, 2020, 1099:136-144. [38] YU L, LAN W, XU H, et al. Label-free detection of Hg2+ based on Hg2+-triggered toehold binding, Exonuclease Ⅲ assisted target recycling and hybridization chain reaction[J]. Sensors and Actuators B:Chemical, 2017, 248:411-418. [39] XU H Y, GENG F H, JIANG X Y, et al. Design of metal-ion-triggered assembly of label-free split G-quadruplex/duplex DNA for turn-on detection of Hg2+ in fetal calf serum[J]. Sensors and Actuators B:Chemical, 2018, 255(1):1024-1030. [40] ZHU Q, LIU L, XING Y, et al. Duplex functional G-quadruplex/NMM fluorescent probe for label-free detection of lead (Ⅱ) and mercury (Ⅱ) ions[J]. Journal of hazardous materials, 2018, 355:50-55. [41] XU M, GAO Z, WEI Q, et al. Label-free hairpin DNA-scaffolded silver nanoclusters for fluorescent detection of Hg2+ using exonuclease Ⅲ-assisted target recycling amplification[J]. Biosensors and Bioelectronics, 2016, 79:411-415. [42] ZHOU D H, ZENG L W, PAN J F, et al. Autocatalytic DNA circuit for Hg2+ detection with high sensitivity and selectivity based on exonuclease Ⅲ and G-quadruplex DNAzyme[J]. Talanta, 2020, 207:120258. [43] 肖志友, 司恒丹, 邓兰清, 等. 基于G-四链体-氯化血红素DNA酶比色法测定银离子和汞离子传感器的构筑[J]. 分析测试学报, 2019, 38(7):840-844. XIAO Z Y, SI H D, DENG L Q, et al. Fabrication of a sensor for colorimetric determination of silver and mercury ions based on G-quadruplex-hemin DNAzymes[J]. Biosensors and Bioelectronics, 2019, 38(7):840-844(in Chinese).
[44] LI T, DONG S, WANG E. Label-free colorimetric detection of aqueous mercury ion (Hg2+) using Hg2+-modulated G-quadruplex-based DNAzymes[J]. Analytical chemistry, 2009, 81(6):2144-2149. [45] JIA S M, LIU X F, LI P, et al. G-quadruplex DNAzyme-based Hg2+ and cysteine sensors utilizing Hg2+-mediated oligonucleotide switching[J]. Biosensors and Bioelectronics, 2011, 27(1):148-152. [46] REN W, ZHANG Y, HUANG W T, et al. Label-free colorimetric detection of Hg2+ based on Hg2+-triggered exonuclease Ⅲ-assisted target recycling and DNAzyme amplification[J]. Biosensors and Bioelectronics, 2015, 68:266-271. [47] ZHANG H, FU X, DENG X, et al. Hg2+-triggered exonuclease Ⅲ-assisted dual-cycle targets recycling amplification for label-free and ultrasensitive colorimetric detection of Hg2+[J]. Sensors and Actuators B:Chemical, 2017, 246:896-903. [48] YUAN J, WU Y, KANG X, et al. Label-free colorimetric detection of divalent mercuric ions (Hg2+) based on T-Hg2+-T structure and exonuclease Ⅲ dual-recycling and G-quadruplex-hemin DNAzyme amplification[J]. International Journal of Environmental Analytical Chemistry, 2019:1-13. [49] LI X Y, DU Z H, LIN S, et al. ExoⅢ and TdT dependent isothermal amplification (ETDA) colorimetric biosensor for ultra-sensitive detection of Hg2+[J]. Food Chemistry, 2020, 316:126303. [50] YU C J, CHENG T L, TSENG W L. Effects of Mn2+ on oligonucleotide-gold nanoparticle hybrids for colorimetric sensing of Hg2+:Improving colorimetric sensitivity and accelerating color change[J]. Biosensors and Bioelectronics, 2009, 25(1):204-210. [51] TAN L, CHEN Z, ZHANG C, et al. Colorimetric detection of Hg2+ based on the growth of aptamer-coated AuNPs:The effect of prolonging aptamer strands[J]. Small, 2017, 13(14):1603370. [52] ZHU Y, CAI Y, ZHU Y, et al. Highly sensitive colorimetric sensor for Hg2+ detection based on cationic polymer/DNA interaction[J]. Biosensors and Bioelectronics, 2015, 69:174-178. [53] YU T, ZHANG T T, ZHAO W, et al. A colorimetric/fluorescent dual-mode sensor for ultra-sensitive detection of Hg2+[J]. Talanta, 2017, 165:570-576. [54] MEMON A G, XING Y, ZHOU X, et al. Ultrasensitive colorimetric aptasensor for Hg2+ detection using Exo-Ⅲ assisted target recycling amplification and unmodified AuNPs as indicators[J]. Journal of Hazardous Materials, 2020, 384:120948. [55] NIU X, DING Y, CHRN C, et al. A novel electrochemical biosensor for Hg2+ determination based on Hg2+-induced DNA hybridization[J]. Sensors & Actuators B Chemical, 2011, 158(1):383-387. [56] LI J, LU L, KANG T, et al. Intense charge transfer surface based on graphene and thymine-Hg(Ⅱ)-thymine base pairs for detection of Hg2+[J]. Biosensors & Bioelectronics, 2016, 77:740-745. [57] LAI Y, MA Y, SUN L, et al. A highly selective electrochemical biosensor for Hg2+ using hemin as a redox indicator[J]. Electrochimica Acta, 2011, 56(9):3153-3158. [58] PARK H, HWANG S J, KIM K. An electrochemical detection of Hg2+ ion using graphene oxide as an electrochemically active indicator[J]. Electrochemistry Communications, 2012, 24(1):100-103. [59] EBRAHIMI M, RAOOF J B, OJANI R, et al. A novel electrochemical biosensor for selective determination of mercury ions based on DNA hybridization[J]. Analytical Biochemistry, 2015, 488:12-13. [60] ZHAO L, WANG Y G, ZHAO G H, et al. Electrochemical aptasensor based on Au@HS-rGO and thymine-Hg2+ -thymine structure for sensitive detection of mercury ion[J]. Journal of Electroanalytical Chemistry, 2019, 848:113308. [61] TORTOLINI C, BOLLELLA P, ANTONELLI M L, et al. DNA-based biosensors for Hg2+ determination by polythymine-methylene blue modified electrodes[J]. Biosensors & Bioelectronics, 2015, 67:524-531. [62] JING J,HONG G C, JI F, et al. A regenerative ratiometric electrochemical biosensor for selective detecting Hg2+ based on Y-shaped/hairpin DNA transformation[J]. Analytica Chimica Acta, 2016, 908:95-101. [63] XIONG E, WU L, ZHOU J, et al. A ratiometric electrochemical biosensor for sensitive detection of Hg2+ based on thymine-Hg2+-thymine structure[J]. Analytica Chimica Acta, 2015, 853:242-248. [64] ZHANG Y Y, CHU G L, GUO Y M, et al. An electrochemical biosensor based on Au nanoparticles decorated reduced graphene oxide for sensitively detecting of Hg2+[J]. Journal of Electroanalytical Chemistry, 2018, 824:201-206. [65] ZENG G, ZHANG C, HUANG D, et al. Practical and regenerable electrochemical aptasensor based on nanoporous gold and thymine-Hg2+-thymine base pairs for Hg2+ detection[J]. Biosensors & Bioelectronics, 2016, 90:542-548. [66] ZHANG Z H, FU X M, LI K Z, et al.One-step fabrication of electrochemical biosensor based on DNA-modified three-dimensional reduced graphene oxide and chitosan nanocomposite for highly sensitive detection of Hg(Ⅱ)[J]. Sensors and Actuators B:Chemical, 2016, 225:453-462. [67] CAO S P, HU H M, LIANG R P, et al. An ultrasensitive electrochemiluminescence resonance energy transfer biosensor for divalent mercury monitoring[J]. Journal of Electroanalytical Chemistry, 2020, 856:113494. [68] HE L L, LIN C, LIN Y, et al. A sensitive biosensor for mercury ions detection based on hairpin hindrance by thymine-Hg(Ⅱ)-thymine structure[J]. Journal of Electroanalytical Chemistry, 2018, 814:161-167. [69] WU S H, ZHANG B, WANG F F, et al. Heating enhanced sensitive and selective electrochemical detection of Hg2+ based on T-Hg2+-T structure and exonuclease Ⅲ-assisted target recycling amplification strategy at heated gold disk electrode[J]. Biosensors and Bioelectronics, 2018, 104:145-151. [70] YU Y J, CHAO Y, GAO R F, et al. Dandelion-like CuO microspheres decorated with Au nanoparticle modified biosensor for Hg2+ detection using a T-Hg2+-T triggered hybridization chain reaction amplification strategy[J]. Biosensors & Bioelectronics, 2019, 131:207-213. [71] HONG M, WANG M, WANG J, et al. Ultrasensitive and selective electrochemical biosensor for detection of mercury (Ⅱ) ions by nicking endonuclease-assisted target recycling and hybridization chain reaction signal amplification[J]. Biosensors & Bioelectronics, 2017, 94:19-23. [72] MEI C Y, LIN D J, FAN C C, et al. Highly sensitive and selective electrochemical detection of Hg2+ through surface-initiated enzymatic polymerization[J]. Biosensors & Bioelectronics, 2016, 80:105-110. -
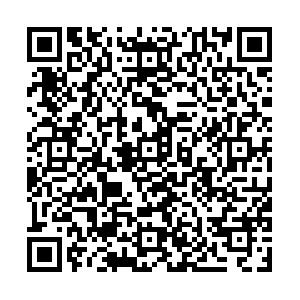
计量
- 文章访问数: 5177
- HTML全文浏览数: 5177
- PDF下载数: 194
- 施引文献: 0