抗生素、重金属和杀生剂抗性共选择机制
Co-selection Mechanism of Antibiotic, Metal and Biocide Resistance
-
摘要: 细菌抗生素抗性已被世界卫生组织认定为对公众健康的重要威胁。细菌抗性除了受到抗生素的直接选择压力,也受到其他物质如重金属和杀生剂的共同影响。研究发现,细菌可以通过外排泵系统、细胞膜通透性改变、作用靶标点的改变、酶修饰或降解作用、应激反应改变生理特性、金属螯合和生物转化等抗性机制对这些选择压力产生抗性,或者通过协同抗性、交叉抗性和共调控抗性的机制产生多重抗性。本文综述了细菌对抗生素、重金属和杀生剂的单一抗性机制、多重抗性机制,以及重金属和杀生剂对细菌抗生素抗性的影响机制,并分析了目前研究的不足之处。Abstract: Bacterial antibiotic resistance has been recognized as an important threat to public health by the World Health Organization. Besides the direct selection pressure of antibiotics, bacterial antibiotic resistance is also affected by some other substances such as heavy metals and biocides. It has been found that the mechanisms of bacterial resistance include efflux pump system, change of cell membrane permeability, change of target sites, modification or degradation of enzymes, change of physiological characteristics by stress response, metal chelation and biotransformation. And multiple resistance could be produced by synergistic, cross-resistance and co-regulation of resistance. In this paper, we summarized the single and multiple resistance mechanisms of bacteria to antibiotics, heavy metals and biocides, and the influence mechanism of heavy metals and biocides on bacterial antibiotic resistance. The short of current researches were also addressed.
-
Key words:
- heavy metal /
- biocide /
- co-resistance /
- cross-resistance /
- co-regulation resistance /
- antibiotic resistance mechanism
-
Davies J, Davies D. Origins and evolution of antibiotic resistance[J]. Microbiología (Madrid, Spain), 1996, 12(1):9-16 Wright G D. Antibiotic resistance in the environment:A link to the clinic?[J]. Current Opinion in Microbiology, 2010, 13(5):589-594 Ying G G, He L Y, Ying A J, et al. China must reduce its antibiotic use[J]. Environmental Science & Technology, 2017, 51(3):1072-1073 Van Boeckel T P, Brower C, Gilbert M, et al. Global trends in antimicrobial use in food animals[J]. Proceedings of the National Academy of Sciences, 2015, 112(18):5649-5654 Port J A, Cullen A C, Wallace J C, et al. Metagenomic frameworks for monitoring antibiotic resistance in aquatic environments[J]. Environmental Health Perspectives, 2014, 122(3):222-228 Zhang Q Q, Ying G G, Pan C G, et al. Comprehensive evaluation of antibiotics emission and fate in the river basins of China:Source analysis, multimedia modeling, and linkage to bacterial resistance[J]. Environmental Science & Technology, 2015, 49(11):6772-6782 World Health Organization. Antimicrobial resistance:Global report on surveillance[J]. Australasian Medical Journal, 2014, 7(4):237[8〛 World Health Organization. Global action plan on antimicrobial resistance 2015[R]. Geneva:World Health Organization, 2015 Baquero F, José-Luis M, Rafael C. Antibiotics and antibiotic resistance in water environments[J]. Current Opinion in Biotechnology, 2008, 19(3):260-265 Chapman J S. Disinfectant resistance mechanisms, crossresistance, and co-resistance[J]. International Biodeterioration and Biodegradation, 2003, 51(4):271-276 Sidhu M S, Heir E, Sørum H, et al. Genetic linkage between resistance to quaternary ammonium compounds and beta-lactam antibiotics in food-related Staphylococcus spp.[J]. Microbial Drug Resistance, 2001, 7(4):363-371 Stepanauskas R, Glenn T C, Jagoe C H, et al. Elevated microbial tolerance to metals and antibiotics in metal-contaminated industrial environments[J]. Environmental Science & Technology, 2005, 39(10):3671-3678 Chapman J S. Biocide resistance mechanisms[J]. International Biodeterioration & Biodegradation, 2003, 51(2):133-138 Bisbiroulas P, Psylou M, Iliopoulou I, et al. Adaptational changes in cellular phospholipids and fatty acid composition of the food pathogen Listeria monocytogenes as a stress response to disinfectant sanitizer benzalkonium chloride[J]. Letters in Applied Microbiology, 2011, 52(3):275-280 Escalada M G. Triclosan inhibition of fatty acid synthesis and its effect on growth of Escherichia coli and Pseudomonas aeruginosa[J]. Journal of Antimicrobial Chemotherapy, 2005, 55(6):879-882 Heath R J, Rubin J R, Holland D R, et al. Mechanism of triclosan inhibition of bacterial fatty acid synthesis[J]. Journal of Biological Chemistry, 1999, 274(16):11110-11114 Meade M J, Waddell R L, Callahan T M. Soil bacteria Pseudomonas putida and Alcaligenes xylosoxidans subsp. denitrificans inactivate triclosan in liquid and solid substrates[J]. FEMS Microbiology Letters, 2001, 204(1):45-48 Nishihara T. Biodegradation of didecyldimethylammonium chloride by Pseudomonas fluorescens TN4 isolated from activated sludge[J]. Journal of Applied Microbiology, 2000, 88(4):641-647 Zhang D F, Li H, Lin X M, et al. Characterization of outer membrane proteins of Escherichia coliin response to phenol stress[J]. Current Microbiology, 2011, 62(3):777-783 Vikram A, Bomberger J M, Bibby K J. Efflux as a glutaraldehyde resistance mechanism in Pseudomonas fluorescens and Pseudomonas aeruginosa biofilms[J]. Antimicrobial Agents and Chemotherapy, 2015, 59(6):3433-3440 Webber M A, Randall L P, Cooles S, et al. Triclosan resistance in Salmonella enterica serovar typhimurium[J]. Journal of Antimicrobial Chemotherapy, 2008, 62(1):92-97 Ortega Morente E, Fernández-Fuentes M A, Grande Burgos M J, et al. Biocide tolerance in bacteria[J]. International Journal of Food Microbiology, 2013, 162(1):13-25 Brown N L, Camakaris J, Lee B T O, et al. Bacterial resistance to mercury and copper[J]. Journal of Cellular Biochemistry, 1991, 46(2):106-114 Han X, Gu J. Sorption and transformation of toxic metals by microorganisms[M]//Mitchell R, Gu J. Environmental Microbiology. Second Edition. John Wiley & Sons, Inc., 2010:153-176 Santiago A G, Chen T Y, Genova L A, et al. Adaptor protein mediates dynamic pump assembly for bacterial metal efflux[J]. Proceedings of the National Academy of Sciences, 2017, 114(26):6694-6699 Baker-Austin C, Wright M S, Stepanauskas R, et al. Coselection of antibiotic and metal resistance[J]. Trends in Microbiology, 2006, 14(4):176-182 Gnanadhas D P, Marathe S A, Chakravortty D. Biocidesresistance, cross-resistance mechanisms and assessment[J]. Expert Opinion on Investigational Drugs, 2013, 22(2):191-206 Pal C, Asiani K, Arya S, et al. Metal resistance and its association with antibiotic resistance[J]. Advances in Microbial Physiology, 2017, 70:261-313 Cheung K C, Poon B H T, Lan C Y, et al. Assessment of metal and nutrient concentrations in river water and sediment collected from the cities in the Pearl River Delta, South China[J]. Chemosphere, 2003, 52(9):1431-1440 Liu W X, Li X D, Shen Z G, et al. Multivariate statistical study of heavy metal enrichment in sediments of the Pearl River Estuary[J]. Environmental Pollution, 2003, 121(3):377-388 吴兴让,尹平河,赵玲,等.珠江广州段微表层和次表层水中重金属分布与风险的初步评价[J].暨南大学学报:自然科学版, 2010, 31(1):84-88 Wu X R, Yin P H, Zhao L, et al. Health risk assessment of heavy metals in the water of surface and subsurface microlayers from Guangzhou section of Pearl River[J]. Journal of Jinan University:Natural Science, 2010, 31(1):84-88(in Chinese)
Zhao J L, Zhang Q Q, Chen F, et al. Evaluation of triclosan and triclocarban at river basin scale using monitoring and modeling tools:Implications for controlling of urban domestic sewage discharge[J]. Water Research, 2013, 47(1):395-405 Rouch D A, Cram D S, Diberardino D, et al. Efflux-mediated antiseptic resistance gene qacA from Staphylococcus aureus:Common ancestry with tetracycline-and sugartransport proteins[J]. Molecular Microbiology, 2010, 4(12):2051-2062 Chopra I. Tetracycline antibiotics:Mode of action, applications, molecular biology, and epidemiology of bacterial resistance[J]. Microbiology and Molecular Biology Reviews, 2001, 65(2):232-260 Schmitz F J, Sadurski R, Kray A, et al. Prevalence of macrolide-resistance genes in Staphylococcus aureus and Enterococcus faecium isolates from 24 European university hospitals[J]. Journal of Antimicrobial Chemotherapy, 2000, 45(6):891-894 Weisblum B. Erythromycin resistance by ribosome modification[J]. Antimicrob Agents and Chemother, 1995, 39(3):577-585 Leclercq R, Courvalin P. Bacterial resistance to macrolide, lincosamide, and streptogramin antibiotics by target modification.[J]. Antimicrobial Agents and Chemotherapy, 1991, 35(7):1267-1272 Cagliero C, Mouline C, Cloeckaert A, et al. Synergy between efflux pump CmeABC and modifications in ribosomal proteins L4 and L22 in conferring macrolide resistance in Campylobacter jejuni and Campylobacter coli[J]. Antimicrobial Agents and Chemotherapy, 2006, 50(11):3893-3896 Muñoz M C C, Benomar N, Ennahar S, et al. Comparative proteomic analysis of a potentially probiotic Lactobacillus pentosus MP-10 for the identification of key proteins involved in antibiotic resistance and biocide tolerance[J]. International Journal of Food Microbiology, 2016, 222:8-15 Chapman J S. Disinfectant resistance mechanisms, crossresistance, and co-resistance[J]. International Biodeterioration and Biodegradation, 2003, 51(4):271-276 Buffet-Bataillon S, Le Jeune A, Le Gall-David S, et al. Molecular mechanisms of higher MICs of antibiotics and quaternary ammonium compounds for Escherichia coli isolated from bacteraemia[J]. Journal of Antimicrobial Chemotherapy, 2012, 67(12):2837-2842 Zhang H, Ma Y, Liu P, et al. Multidrug resistance operon emrAB contributes for chromate and ampicillin co-resistance in a Staphylococcus strain isolated from refinery polluted river bank[J]. SpringerPlus, 2016, 5(1):1648 Fang L, Li X, Li L, et al. Co-spread of metal and antibiotic resistance within ST3-IncHI2 plasmids from E. coli isolates of food-producing animals[J]. Scientific Reports, 2016, 6:25312 Sandegren L, Linkevicius M, Lytsy B, et al. Transfer of an Escherichia coli ST131 multiresistance cassette has created a Klebsiella pneumoniae-specific plasmid associated with a major nosocomial outbreak[J]. Journal of Antimicrobial Chemotherapy, 2011, 67(1):74-83 Schwaiger K, Harms K S, Bischoff M, et al. Insusceptibility to disinfectants in bacteria from animals, food and humans-Is there a link to antimicrobial resistance?[J]. Frontiers in Microbiology, 2014, 5:88 Chuanchuen R, Beinlich K, Hoang T T, et al. Cross-resistance between triclosan and antibiotics in Pseudomonas aeruginosa is mediated by multidrug efflux pumps:Exposure of a susceptible mutant strain to triclosan selects nfxB mutants overexpressing MexCD-OprJ[J]. Antimicrobial Agents and Chemotherapy, 2001, 45(2):428-432 Webber M A, Buckner M M C, Redgrave L S, et al. Quinolone-resistant gyrase mutants demonstrate decreased susceptibility to triclosan[J]. Journal of Antimicrobial Chemotherapy, 2017, 72(10):2755-2763 Lin J, Michel L O, Zhang Q. CmeABC functions as a multidrug efflux system in Campylobacter jejuni[J]. Antimicrobial Agents and Chemotherapy, 2002, 46(7):2124-2131 Pumbwe L, Randall L P, Woodward M J, et al. Evidence for multiple-antibiotic resistance in Campylobacter jejuni not mediated by CmeB or CmeF[J]. Antimicrobial Agents and Chemotherapy, 2005, 49(4):1289-1293 Conroy O, Kim E H, Mcevoy M M, et al. Differing ability to transport nonmetal substrates by two RND-type metal exporters[J]. FEMS Microbiology Letters, 2010, 308(2):115-122 Schweizer H P. Intrinsic resistance to inhibitors of fatty acid biosynthesis in Pseudomonas aeruginosa is due to efflux:Application of a novel technique for generation of unmarked chromosomal mutations for the study of efflux systems[J]. Antimicrobial Agents and Chemotherapy, 1998, 42(2):394-398 Nishino K, Nikaido E, Yamaguchi A. Regulation of multidrug efflux systems involved in multidrug and metal resistance of Salmonella enterica serovar Typhimurium[J]. Journal of Bacteriology, 2007, 189(24):9066-9075 Shahcheraghi F, Minato Y, Chen J, et al. Molecular cloning and characterization of a multidrug efflux pump, SmfY, from Serratia marcescens[J]. Biological and Pharmaceutical Bulletin, 2007, 30(4):798-800 Langsrud S, Sundheim G, Holck A L. Cross-resistance to antibiotics of Escherichia coli adapted to benzalkonium chloride or exposed to stress-inducers[J]. Journal of Applied Microbiology, 2004, 96(1):201-208 Navarre W W, Halsey T A, Walthers D, et al. Co-regulation of Salmonella enterica genes required for virulence and resistance to antimicrobial peptides by SlyA and PhoP/PhoQ[J]. Molecular Microbiology, 2005, 56(2):492-508 Perron K, Caille O, Rossier C, et al. CzcR-CzcS, a twocomponent system involved in heavy metal and carbapenem resistance in Pseudomonas aeruginosa[J]. Journal of Biological Chemistry, 2004, 279(10):8761-8768 Balasubramanian D, Kong K F, Jayawardena S R, et al. Co-regulation of β-lactam resistance, alginate production and quorum sensing in Pseudomonas aeruginosa[J]. Journal of Medical Microbiology, 2011, 60(2):147-156 Lu J, Jin M, Nguyen S H, et al. Non-antibiotic antimicrobial triclosan induces multiple antibiotic resistance through genetic mutation[J]. Environment International, 2018, 118:257-265 Chen S, Li X, Sun G, et al. Heavy metal induced antibiotic resistance in bacterium LSJC7[J]. International Journal of Molecular Sciences, 2015, 16(10):23390-23404 Flach C F, Pal C, Svensson C J, et al. Does antifouling paint select for antibiotic resistance?[J]. Science of the Total Environment, 2017, 590:461-468 Zhang M, Chen L, Ye C, et al. Co-selection of antibiotic resistance via copper shock loading on bacteria from a drinking water bio-filter[J]. Environmental Pollution, 2018, 233:132-141 Xu Y, Xu J, Mao D, et al. Effect of the selective pressure of sub-lethal level of heavy metals on the fate and distribution of ARGs in the catchment scale[J]. Environmental Pollution, 2017, 220:900-908 Kang W, Zhang Y J, Shi X, et al. Short-term copper exposure as a selection pressure for antibiotic resistance and metal resistance in an agricultural soil[J]. Environmental Science and Pollution Research, 2018, 25(29):29314-29324 Zhang S, Wang Y, Song H, et al. Copper nanoparticles and copper ions promote horizontal transfer of plasmidmediated multi-antibiotic resistance genes across bacterial genera[J]. Environment International, 2019, 129:478-487 Zhang Y, Gu A Z, Cen T, et al. Sub-inhibitory concentrations of heavy metals facilitate the horizontal transfer of plasmid-mediated antibiotic resistance genes in water environment[J]. Environmental Pollution, 2018, 237:74-82 Rizzotti L, Rossi F, Torriani S. Biocide and antibiotic resistance of Enterococcus faecalis and Enterococcus faecium isolated from the swine meat chain[J]. Food Microbiology, 2016, 60:160-164 Khan S, Beattie T K, Knapp C W. Relationship between antibiotic-and disinfectant-resistance profiles in bacteria harvested from tap water[J]. Chemosphere, 2016, 152:132-141 Oggioni M R, Coelho J R, Furi L, et al. Significant differences characterise the correlation coefficients between biocide and antibiotic susceptibility profiles in Staphylococcus aureus[J]. Current Pharmaceutical Design, 2015, 21(16):2054 Fahimipour A K, Ben Mamaar S, Mcfarland A G, et al. Antimicrobial chemicals associate with microbial function and antibiotic resistance indoors[J]. mSystems, 2018, 3(6):e00200-18 Liu S S, Qu H M, Yang D, et al. Chlorine disinfection increases both intracellular and extracellular antibiotic resistance genes in a full-scale wastewater treatment plant[J]. Water Research, 2018, 136:131-136 Hernández A, Ruiz F M, Romero A, et al. The binding of triclosan to SmeT, the repressor of the multidrug efflux pump SmeDEF, induces antibiotic resistance in Stenotrophomonas maltophilia[J]. PLoS Pathogens (Online), 2011, 7(6):e1002103 Webber M A, Whitehead R N, Mount M, et al. Parallel evolutionary pathways to antibiotic resistance selected by biocide exposure[J]. Journal of Antimicrobial Chemotherapy, 2015, 70(8):2241-2248 Li D, Zeng S, He M, et al. Water disinfection byproducts induce antibiotic resistance-role of environmental pollutants in resistance phenomena[J]. Environmental Science & Technology, 2016, 50(6):3193-3201 Li M, He Y, Sun J, et al. Chronic exposure to an environmentally relevant triclosan concentration induces persistent triclosan resistance but reversible antibiotic tolerance in Escherichia coli[J]. Environmental Science & Technology, 2019, 53(6):3277-3286 Jutkina J, Marathe N P, Flach C F, et al. Antibiotics and common antibacterial biocides stimulate horizontal transfer of resistance at low concentrations[J]. Science of the Total Environment, 2018, 616:172-178 -
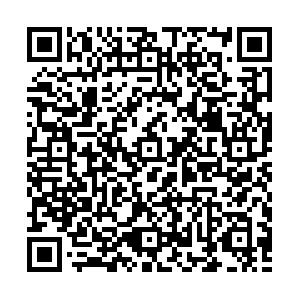
计量
- 文章访问数: 9110
- HTML全文浏览数: 9110
- PDF下载数: 353
- 施引文献: 0