纳米零价铁的表征及改性研究进展
Research progress on the characterization and modification of nanoscale zero-valent iron for applications
-
摘要: 纳米零价铁(nanoscale zero-valent iron,nZVI)因其在地下水处理和土壤修复中具有应用前景一直广受关注.但在实际应用中,纳米零价铁nZVI依旧存在颗粒易团聚、传输能力弱和污染物难以选择性去除等方面的问题.为突破这些应用瓶颈,目前已开展了大量nZVI改性工作,并对污染物去除机理进行了深入研究.相关研究很大程度上解决了目前nZVI在环境应用中存在的缺陷,大幅提升了nZVI的环境修复效率.本文归纳了近年来nZVI颗粒在机理研究和性能优化中涉及的表征方法,详细介绍了nZVI表面改性技术(金属掺杂、表面涂层、乳化和负载等)及其优势.同时,展望了nZVI在实际应用中面临的科学挑战与发展机遇,其有助于学者们对nZVI的相关应用有更深的理解,有望为nZVI等环境纳米材料实际应用提供相关借鉴.Abstract: Nanoscale zero-valent iron(nZVI) has received considerable attention due to its potential applications in groundwater treatment and site remediation. The current field application of nZVI is constrained by the problems of agglomeration of particles, poor transmission efficiency, and low selectivity of pollutants. Considering the above-mentioned bottlenecks, the research on surface modification is expected to improve the dispersibility, mechanical strength and reactivity of nZVI, thus greatly improving the environmental remediation efficiency. This article reviews the recent research progress of nZVI characterization methods to accurately reveal the mechanisms on its interaction with pollutants, and summarizes the nZVI surface modification technologies (including metal doping, surface coating, emulsification and supporting). Furthermore, the scientific challenges and future development opportunities of nZVI in practical application are also prospected.
-
-
[1] WANG C B,ZHANG W X. Synthesizing nanoscale iron particles for rapid and complete dechlorination of TCE and PCBs[J]. Environmental Science & Technology, 1997, 31:2154-2156. [2] LI S, WANG W, LIANG F, et al. Heavy metal removal using nanoscale zero-valent iron (nZVI):Theory and application[J]. Journal of Hazardous Materials, 2017, 322:163-171. [3] MUELLER N C, BRAUN J, BRUNS J, et al. Application of nanoscale zero valent iron (NZVI) for groundwater remediation in Europe[J]. Environmental Science and Pollution Research, 2012, 19:550-558. [4] WEI Y T, WU S C, CHOU C M, et al. Influence of nanoscale zero-valent iron on geochemical properties of groundwater and vinyl chloride degradation:A field case study[J]. Water Research, 2010, 44:131-140. [5] FU F, DIONYSIOU D D,LIU H. The use of zero-valent iron for groundwater remediation and wastewater treatment:A review[J]. Journal of Hazardous Materials, 2014, 267:194-205. [6] SIGNORINI L, PASQUINI L, SAVINI L, et al. Size-dependent oxidation in iron/iron oxide core-shell nanoparticles[J]. Physical Review B, 2003, 68:195423. [7] WANG C, BAER D R, AMONETTE J E, et al. Morphology and electronic structure of the oxide shell on the surface of iron nanoparticles[J]. Journal of the American Chemical Society, 2009, 131:8824-8832. [8] YAN W, VASIC R, FRENKEL A I, et al. Intraparticle reduction of arsenite (As(Ⅲ)) by nanoscale zerovalent iron (nZVI) investigated with In situ X-ray absorption spectroscopy[J]. Environmental Science & Technology, 2012, 46:7018-7026. [9] YAN W, HERZING A A, KIELY C J, et al. Nanoscale zero-valent iron (nZVI):Aspects of the core-shell structure and reactions with inorganic species in water[J]. Journal of Contaminant Hydrology, 2010, 118:96-104. [10] 黄潇月, 王伟, 凌岚, 等.纳米零价铁与重金属的反应:"核-壳"结构在重金属去除中的作用[J]. 化学学报. 2017, 75(6):529-537. HUANG X Y, WANG W, LING L, et al. Heavy metal-nZVI reactions:The core-shell structure and applications for heavy metal treatment[J]. Acta Chim. Sinica, 2017, 75(6):529-537(in Chinese)
[11] LING L, PAN B,ZHANG W X. Removal of selenium from water with nanoscale zero-valent iron:Mechanisms of intraparticle reduction of Se(Ⅳ)[J]. Water Research, 2015, 71:274-281. [12] 李钰婷, 张亚雷, 代朝猛, 等.纳米零价铁颗粒去除水中重金属的研究进展[J]. 环境化学, 2012, 31(9):1349-1354. LI Y T, ZHANG Y L, DAI C M, et al. The advance on removal of heavy metals in water by nanoscale zero-valent iron[J]. Environmental Chemistry, 2012, 31(9):1349-1354(in Chinese).
[13] 夏雪芬, 滑熠龙, 黄潇月, 等.纳米零价铁对水中砷和硒去除的比较研究[J]. 化学学报, 2017, 75(6):594-601. XIA X F, HUA Y L, HUANG X Y, et al. Removal of arsenic and selenium with nanoscale zero-valent iron (nZVI)[J]. Acta Chim Sinica, 2017, 75(6):594-601(in Chinese).
[14] LING L, HUANG X Y,ZHANG W X. Enrichment of precious metals from wastewater with core-shell nanoparticles of iron[J]. Advanced Materials, 2018, 30:1705703. [15] LING L, TANG C,ZHANG W X. Visualization of silver nanoparticle formation on nanoscale zero-valent iron[J]. Environmental Science & Technology Letters, 2018, 5:520-525. [16] LIU Z, DONG S, ZOU D, et al. Electrochemically mediated nitrate reduction on nanoconfined zerovalent iron:Properties and mechanism[J]. Water Research, 2020, 173:115596. [17] KATSOYIANNIS I A, RUETTIMANN T,HUG S J. pH dependence of fenton reagent generation and As(Ⅲ) oxidation and removal by corrosion of zero valent iron in aerated water[J]. Environmental Science & Technology, 2008, 42:7424-7430. [18] SEDLAK D L,ANDREN A W. Oxidation of chlorobenzene with Fenton's reagent[J]. Environmental Science & Technology, 1991, 25:777-782. [19] SHI Z, FAN D, JOHNSON R L, et al. Methods for characterizing the fate and effects of nano zerovalent iron during groundwater remediation[J]. Journal of Contaminant Hydrology, 2015, 181:17-35. [20] SU Y, ADELEYE A S, KELLER A A, et al. Magnetic sulfide-modified nanoscale zerovalent iron (S-nZVI) for dissolved metal ion removal[J]. Water Research, 2015, 74:47-57. [21] SHARD A G. A straightforward method for interpreting XPS data from core-shell nanoparticles[J]. The Journal of Physical Chemistry C, 2012, 116:16806-16813. [22] XI Y, MALLAVARAPU M,NAIDU R. Reduction and adsorption of Pb2+ in aqueous solution by nano-zero-valent iron-A SEM, TEM and XPS study[J]. Materials Research Bulletin, 2010, 45:1361-1367. [23] KUMAR N, AUFFAN M, GATTACCECA J, et al. Molecular insights of oxidation process of iron nanoparticles:Spectroscopic, magnetic, and microscopic evidence[J]. Environmental Science & Technology, 2014, 48:13888-13894. [24] WANG Q, SNYDER S, KIM J, et al. Aqueous ethanol modified nanoscale zerovalent iron in bromate reduction:Synthesis, characterization, and reactivity[J]. Environmental Science & Technology, 2009, 43:3292-3299. [25] YAN W, LIEN H L, KOEL B E, et al. Iron nanoparticles for environmental clean-up:Recent developments and future outlook[J]. Environmental Science:Processes & Impacts, 2013, 15:63-77. [26] WANG C M, BAER D R, THOMAS L E, et al. Void formation during early stages of passivation:Initial oxidation of iron nanoparticles at room temperature[J]. Journal of Applied Physics, 2005, 98:094308. [27] NURMI J T, TRATNYEK P G, SARATHY V, et al. Characterization and properties of metallic iron nanoparticles:Spectroscopy, electrochemistry, and kinetics[J]. Environmental Science & Technology, 2005, 39:1221-1230. [28] LIU Y, CHOI H, DIONYSIOU D, et al. Trichloroethene hydrodechlorination in water by highly disordered monometallic nanoiron[J]. Chemistry of Materials, 2005, 17:5315-5322. [29] LING L, HUANG X, LI M, et al. Mapping the reactions in a single zero-valent iron nanoparticle[J]. Environmental Science & Technology, 2017, 51:14293-14300. [30] LING L,ZHANG W X. Enrichment and encapsulation of uranium with iron nanoparticle[J]. Journal of the American Chemical Society, 2015, 137:2788-2791. [31] LING L,ZHANG W X. Visualizing arsenate reactions and encapsulation in a single zero-valent iron nanoparticle[J]. Environmental Science & Technology, 2017, 51:2288-2294. [32] MARTIN J E, HERZING A A, YAN W, et al. Determination of the oxide layer thickness in core-shell zerovalent iron nanoparticles[J]. Langmuir, 2008, 24:4329-4334. [33] MU Y, JIA F, AI Z, et al. Iron oxide shell mediated environmental remediation properties of nano zero-valent iron[J]. Environmental Science:Nano, 2017, 4:27-45. [34] SUN Y P, LI X Q, CAO J, et al. Characterization of zero-valent iron nanoparticles[J]. Advances in Colloid and Interface Science, 2006, 120:47-56. [35] COLLIEX C, MANOUBI T,ORTIZ C. Electron-energy-loss-spectroscopy near-edge fine structures in the iron-oxygen system[J]. Physical Review B, 1991, 44:11402-11411. [36] STEFANIUK M, OLESZCZUK P,OK Y S. Review on nano zerovalent iron (nZVI):From synthesis to environmental applications[J]. Chemical Engineering Journal, 2016, 287:618-632. [37] GLAVEE G N, KLABUNDE K J, SORENSEN C M, et al. Chemistry of borohydride reduction of iron(Ⅱ) and iron(Ⅲ) ions in aqueous and nonaqueous media. formation of nanoscale Fe, FeB, and Fe2B powders[J]. Inorganic Chemistry, 1995, 34:28-35. [38] HAHN H. Gas phase synthesis of nanocrystalline materials[J]. Nanostructured Materials, 1997, 9:3-12. [39] CARPENTER E E, CALVIN S, STROUD R M, et al. Passivated iron as core-shell nanoparticles[J]. Chemistry of Materials, 2003, 15:3245-3246. [40] LI F, VIPULANANDAN C,MOHANTY K K. Microemulsion and solution approaches to nanoparticle iron production for degradation of trichloroethylene[J]. Colloids and Surfaces A:Physicochemical and Engineering Aspects, 2003, 223:103-112. [41] SHAN G, YAN S, TYAGI R D, et al. Applications of nanomaterials in environmental science and engineering:Review[J]. Practice Periodical of Hazardous, Toxic, and Radioactive Waste Management, 2009, 13:110-119. [42] LI S, YAN W,ZHANG W X. Solvent-free production of nanoscale zero-valent iron (nZVI) with precision milling[J]. Green Chemistry, 2009, 11:1618-1626. [43] 杜毅, 王向宇. 新型纳米零价铁的绿色合成和改性工艺研究进展[J]. 环境化学, 2016, 35(2):337-347. DU Y, WANG X Y. Green synthesis and modification of nano zero-valent iron[J]. Environmental Chemistry, 2016, 35(2):337-347(in Chinese).
[44] HOAG G E, COLLINS J B, HOLCOMB J L, et al. Degradation of bromothymol blue by ‘greener’ nano-scale zero-valent iron synthesized using tea polyphenols[J]. Journal of Materials Chemistry, 2009, 19:8671-8677. [45] NJAGI E C, HUANG H, STAFFORD L, et al. Biosynthesis of iron and silver nanoparticles at room temperature using aqueous sorghum bran extracts[J]. Langmuir, 2011, 27:264-271. [46] WENG X, GUO M, LUO F, et al. One-step green synthesis of bimetallic Fe/Ni nanoparticles by eucalyptus leaf extract:Biomolecules identification, characterization and catalytic activity[J]. Chemical Engineering Journal, 2017, 308:904-911. [47] MACHADO S, GROSSO J P, NOUWS H P A, et al. Utilization of food industry wastes for the production of zero-valent iron nanoparticles[J]. Science of the Total Environment, 2014, 496:233-240. [48] ZHANG W X, WANG C B,LIEN H L. Treatment of chlorinated organic contaminants with nanoscale bimetallic particles[J]. Catalysis Today, 1998, 40:387-395. [49] LIEN H L,ZHANG W X. Hydrodechlorination of chlorinated ethanes by nanoscale Pd/Fe bimetallic particles[J]. Journal of Environmental Engineering, 2005, 131:4-10. [50] XU Y,ZHANG W X. Subcolloidal Fe/Ag particles for reductive dehalogenation of chlorinated benzenes[J]. Industrial & Engineering Chemistry Research, 2000, 39:2238-2244. [51] SCHRICK B, BLOUGH J L, JONES A D, et al. Hydrodechlorination of trichloroethylene to hydrocarbons using bimetallic nickel-iron nanoparticles[J]. Chemistry of Materials, 2002, 14:5140-5147. [52] BARNES R J, RIBA O, GARDNER M N, et al. Optimization of nano-scale nickel/iron particles for the reduction of high concentration chlorinated aliphatic hydrocarbon solutions[J]. Chemosphere, 2010, 79:448-454. [53] LAI B, ZHANG Y, CHEN Z, et al. Removal of p-nitrophenol (PNP) in aqueous solution by the micron-scale iron-copper (Fe/Cu) bimetallic particles[J]. Applied Catalysis B:Environmental, 2014, 144:816-830. [54] YAN W, HERZING A A, LI X Q, et al. Structural evolution of Pd-doped nanoscale zero-valent iron (nZVI) in aqueous media and implications for particle aging and reactivity[J]. Environmental Science & Technology, 2010, 44:4288-4294. [55] SUN Y P, LI X Q, ZHANG W X, et al. A method for the preparation of stable dispersion of zero-valent iron nanoparticles[J]. Colloids and Surfaces A:Physicochemical and Engineering Aspects, 2007, 308:60-66. [56] PHENRAT T, SALEH N, SIRK K, et al. Aggregation and sedimentation of aqueous nanoscale zerovalent iron dispersions[J]. Environmental Science & Technology, 2007, 41:284-290. [57] SCHRICK B, HYDUTSKY B W, BLOUGH J L, et al. Delivery vehicles for zerovalent metal nanoparticles in soil and groundwater[J]. Chemistry of Materials, 2004, 16:2187-2193. [58] ZOU Y, WANG X, KHAN A, et al. Environmental remediation and application of nanoscale zero-valent iron and its composites for the removal of heavy metal ions:A review[J]. Environmental Science & Technology, 2016, 50:7290-7304. [59] SALEH N, SARBU T, SIRK K, et al. Oil-in-water emulsions stabilized by highly charged polyelectrolyte-grafted silica nanoparticles[J]. Langmuir, 2005, 21:9873-9878. [60] SIRK K M, SALEH N B, PHENRAT T, et al. Effect of adsorbed polyelectrolytes on nanoscale zero valent iron particle attachment to soil surface models[J]. Environmental Science & Technology, 2009, 43:3803-3808. [61] JIEMVARANGKUL P, ZHANG W X,LIEN H L. Enhanced transport of polyelectrolyte stabilized nanoscale zero-valent iron (nZVI) in porous media[J]. Chemical Engineering Journal, 2011, 170:482-491. [62] HE F,ZHAO D. Manipulating the size and dispersibility of zerovalent iron nanoparticles by use of carboxymethyl cellulose stabilizers[J]. Environmental Science & Technology, 2007, 41:6216-6221. [63] KANEL S R, GOSWAMI R R, CLEMENT T P, et al. Two dimensional transport characteristics of surface stabilized zero-valent iron nanoparticles in porous media[J]. Environmental Science & Technology, 2008, 42:896-900. [64] PHENRAT T, SALEH N, SIRK K, et al. Stabilization of aqueous nanoscale zerovalent iron dispersions by anionic polyelectrolytes:Adsorbed anionic polyelectrolyte layer properties and their effect on aggregation and sedimentation[J]. Journal of Nanoparticle Research, 2008, 10:795-814. [65] PHENRAT T, KIM H J, FAGERLUND F, et al. Particle size distribution, concentration, and magnetic attraction affect transport of polymer-modified Fe0 nanoparticles in sand columns[J]. Environmental Science & Technology, 2009, 43:5079-5085. [66] PHENRAT T, FAGERLUND F, ILLANGASEKARE T, et al. Polymer-modified Fe0 nanoparticles target entrapped NAPL in two dimensional porous media:Effect of particle concentration, NAPL saturation, and injection strategy[J]. Environmental Science & Technology, 2011, 45:6102-6109. [67] TIRAFERRI A, CHEN K L, SETHI R, et al. Reduced aggregation and sedimentation of zero-valent iron nanoparticles in the presence of guar gum[J]. Journal of Colloid and Interface Science, 2008, 324:71-79. [68] XIONG Z, ZHAO D,PAN G. Rapid and complete destruction of perchlorate in water and ion-exchange brine using stabilized zero-valent iron nanoparticles[J]. Water Research, 2007, 41:3497-3505. [69] COMBA S,SETHI R. Stabilization of highly concentrated suspensions of iron nanoparticles using shear-thinning gels of xanthan gum[J]. Water Research, 2009, 43:3717-3726. [70] QIU X, FANG Z, YAN X, et al. Emergency remediation of simulated chromium (Ⅵ)-polluted river by nanoscale zero-valent iron:Laboratory study and numerical simulation[J]. Chemical Engineering Journal, 2012, 193/194:358-365. [71] LAUMANN S, MICIĆ V,HOFMANN T. Mobility enhancement of nanoscale zero-valent iron in carbonate porous media through co-injection of polyelectrolytes[J]. Water Research, 2014, 50:70-79. [72] LIN Y H, TSENG H H, WEY M Y, et al. Characteristics of two types of stabilized nano zero-valent iron and transport in porous media[J]. Science of the Total Environment, 2010, 408:2260-2267. [73] LV D, ZHOU J, CAO Z, et al. Mechanism and influence factors of chromium(Ⅵ) removal by sulfide-modified nanoscale zerovalent iron[J]. Chemosphere, 2019, 224:306-315. [74] RAJAJAYAVEL S R C,GHOSHAL S. Enhanced reductive dechlorination of trichloroethylene by sulfidated nanoscale zerovalent iron[J]. Water Research, 2015, 78:144-153. [75] XU J, AVELLAN A, LI H, et al. Sulfur loading and speciation control the hydrophobicity, electron transfer, reactivity, and selectivity of sulfidized nanoscale zerovalent iron[J]. Advanced Materials, 2020, 32:1906910. [76] KIM E J, KIM J H, CHANG Y S, et al. Effects of metal ions on the reactivity and corrosion electrochemistry of Fe/FeS nanoparticles[J]. Environmental Science & Technology, 2014, 48:4002-4011. [77] SU Y, JASSBY D, SONG S, et al. Enhanced oxidative and adsorptive removal of diclofenac in heterogeneous fenton-like reaction with sulfide modified nanoscale zerovalent iron[J]. Environmental Science & Technology, 2018, 52:6466-6475. [78] GU Y, WANG B, HE F, et al. Mechanochemically sulfidated microscale zero valent iron:pathways, kinetics, mechanism, and efficiency of trichloroethylene dechlorination[J]. Environmental Science & Technology, 2017, 51:12653-12662. [79] QUINN J, GEIGER C, CLAUSEN C, et al. Field demonstration of DNAPL dehalogenation using emulsified zero-valent iron[J]. Environmental Science & Technology, 2005, 39:1309-1318. [80] BERGE N D,RAMSBURG C A. Oil-in-water emulsions for encapsulated delivery of reactive iron particles[J]. Environmental Science & Technology, 2009, 43:5060-5066. [81] YAN J, HAN L, GAO W, et al. Biochar supported nanoscale zerovalent iron composite used as persulfate activator for removing trichloroethylene[J]. Bioresource Technology, 2015, 175:269-274. [82] ZHU H, JIA Y, WU X, et al. Removal of arsenic from water by supported nano zero-valent iron on activated carbon[J]. Journal of Hazardous Materials, 2009, 172:1591-1596. [83] SUN Y, DING C, CHENG W, et al. Simultaneous adsorption and reduction of U(Ⅵ) on reduced graphene oxide-supported nanoscale zerovalent iron[J]. Journal of Hazardous Materials, 2014, 280:399-408. [84] DU Q, ZHANG S, PAN B, et al. Bifunctional resin-ZVI composites for effective removal of arsenite through simultaneous adsorption and oxidation[J]. Water Research, 2013, 47:6064-6074. [85] LI A, TAI C, ZHAO Z, et al. Debromination of decabrominated diphenyl ether by resin-bound iron nanoparticles[J]. Environmental Science & Technology, 2007, 41:6841-6846. [86] ZHANG R, LI J, LIU C, et al. Reduction of nitrobenzene using nanoscale zero-valent iron confined in channels of ordered mesoporous silica[J]. Colloids and Surfaces A:Physicochemical and Engineering Aspects, 2013, 425:108-114. [87] SUN X, YAN Y, LI J, et al. SBA-15-incorporated nanoscale zero-valent iron particles for chromium(Ⅵ) removal from groundwater:Mechanism, effect of pH, humic acid and sustained reactivity[J]. Journal of Hazardous Materials, 2014, 266:26-33. [88] HU B, CHEN G, JIN C, et al. Macroscopic and spectroscopic studies of the enhanced scavenging of Cr(Ⅵ) and Se(Ⅵ) from water by titanate nanotube anchored nanoscale zero-valent iron[J]. Journal of Hazardous Materials, 2017, 336:214-221. [89] SHENG G, ALSAEDI A, SHAMMAKH W, et al. Enhanced sequestration of selenite in water by nanoscale zero valent iron immobilization on carbon nanotubes by a combined batch, XPS and XAFS investigation[J]. Carbon, 2016, 99:123-130. [90] LIU M, WANG Y, CHEN L, et al. Mg(OH)2 supported nanoscale zero valent iron enhancing the removal of Pb(Ⅱ) from aqueous solution[J]. ACS Applied Materials & Interfaces, 2015, 7:7961-7969. [91] LIU T, YANG Y, WANG Z L, et al. Remediation of arsenic(Ⅲ) from aqueous solutions using improved nanoscale zero-valent iron on pumice[J]. Chemical Engineering Journal, 2016, 288:739-744. [92] LIU T, WANG Z L, YAN X, et al. Removal of mercury (Ⅱ) and chromium (Ⅵ) from wastewater using a new and effective composite:Pumice-supported nanoscale zero-valent iron[J]. Chemical Engineering Journal, 2014, 245:34-40. [93] ZHANG X, LIN S, CHEN Z, et al. Kaolinite-supported nanoscale zero-valent iron for removal of Pb2+ from aqueous solution:Reactivity, characterization and mechanism[J]. Water Research, 2011, 45:3481-3488. [94] ARANCIBIA-MIRANDA N, BALTAZAR S E, GARCíA A, et al. Nanoscale zero valent supported by zeolite and montmorillonite:Template effect of the removal of lead ion from an aqueous solution[J]. Journal of Hazardous Materials, 2016, 301:371-380. [95] LI Z, WANG L, MENG J, et al. Zeolite-supported nanoscale zero-valent iron:New findings on simultaneous adsorption of Cd(Ⅱ), Pb(Ⅱ), and As(Ⅲ) in aqueous solution and soil[J]. Journal of Hazardous Materials, 2018, 344:1-11. [96] HU B, YE F, REN X, et al. X-ray absorption fine structure study of enhanced sequestration of U(Ⅵ) and Se(Ⅳ) by montmorillonite decorated with zero-valent iron nanoparticles[J]. Environmental Science:Nano, 2016, 3:1460-1472. [97] GU C, JIA H, LI H, et al. Synthesis of highly reactive subnano-sized zero-valent iron using smectite clay templates[J]. Environmental Science & Technology, 2010, 44:4258-4263. [98] YUAN N, ZHANG G, GUO S, et al. Enhanced ultrasound-assisted degradation of methyl orange and metronidazole by rectorite-supported nanoscale zero-valent iron[J]. Ultrasonics Sonochemistry, 2016, 28:62-68. [99] FU R, YANG Y, XU Z, et al. The removal of chromium (Ⅵ) and lead (Ⅱ) from groundwater using sepiolite-supported nanoscale zero-valent iron (S-NZVI)[J]. Chemosphere, 2015, 138:726-734. [100] YUAN C,LIEN H L. Removal of arsenate from aqueous solution using nanoscale iron particles[J]. Water Quality Research Journal, 2006, 41:210-215. [101] ÇELEBI O, ÜZüM Ç, SHAHWAN T, et al. A radiotracer study of the adsorption behavior of aqueous Ba2+ ions on nanoparticles of zero-valent iron[J]. Journal of Hazardous Materials, 2007, 148:761-767. [102] ÜZüM Ç, SHAHWAN T, EROǦLU A E, et al. Application of zero-valent iron nanoparticles for the removal of aqueous Co2+ ions under various experimental conditions[J]. Chemical Engineering Journal, 2008, 144:213-220. [103] BOPARAI H K, JOSEPH M,O'CARROLL D M. Kinetics and thermodynamics of cadmium ion removal by adsorption onto nano zerovalent iron particles[J]. Journal of Hazardous Materials, 2011, 186:458-465. [104] HUANG P, YE Z, XIE W, et al. Rapid magnetic removal of aqueous heavy metals and their relevant mechanisms using nanoscale zero valent iron (nZVI) particles[J]. Water Research, 2013, 47:4050-4058. [105] ARANCIBIA-MIRANDA N, BALTAZAR S E, GARCíA A, et al. Lead removal by nano-scale zero valent iron:Surface analysis and pH effect[J]. Materials Research Bulletin, 2014, 59:341-348. [106] HABISH A J, LAZAREVIĆ S, JANKOVIĆ-ČASTVAN I, et al. Nanoscale zerovalent iron (nZVI) supported by natural and acid-activated sepiolites:The effect of the nZVI/support ratio on the composite properties and Cd2+ adsorption[J]. Environmental Science and Pollution Research, 2017, 24:628-643. [107] WANG C, LUO H, ZHANG Z, et al. Removal of As(Ⅲ) and As(Ⅴ) from aqueous solutions using nanoscale zero valent iron-reduced graphite oxide modified composites[J]. Journal of Hazardous Materials, 2014, 268:124-131. [108] XING M,WANG J. Nanoscaled zero valent iron/graphene composite as an efficient adsorbent for Co(Ⅱ) removal from aqueous solution[J]. Journal of Colloid and Interface Science, 2016, 474:119-128. [109] SHARMA A K, KUMAR R, MITTAL S, et al. In situ reductive regeneration of zerovalent iron nanoparticles immobilized on cellulose for atom efficient Cr(Ⅵ) adsorption[J]. RSC Advances, 2015, 5:89441-89446. [110] LI Z, DONG H, ZHANG Y, et al. Enhanced removal of Ni(Ⅱ) by nanoscale zero valent iron supported on Na-saturated bentonite[J]. Journal of Colloid and Interface Science, 2017, 497:43-49. [111] ÜZÜM Ç, SHAHWAN T, EROǦLU A E, et al. Synthesis and characterization of kaolinite-supported zero-valent iron nanoparticles and their application for the removal of aqueous Cu2+ and Co2+ ions[J]. Applied Clay Science, 2009, 43:172-181. [112] WANG J, LIU G, LI T, et al. Zero-valent iron nanoparticles (nZVI) supported by kaolinite for Cu(Ⅱ) and Ni(Ⅱ) ion removal by adsorption:Kinetics, thermodynamics, and mechanism[J]. Australian Journal of Chemistry, 2015, 68:1305-1315. [113] WANG Q, QIAN H, YANG Y, et al. Reduction of hexavalent chromium by carboxymethyl cellulose-stabilized zero-valent iron nanoparticles[J]. Journal of Contaminant Hydrology, 2010, 114:35-42. [114] SHI L N, ZHANG X,CHEN Z L. Removal of Chromium (Ⅵ) from wastewater using bentonite-supported nanoscale zero-valent iron[J]. Water Research, 2011, 45:886-892. [115] ZHANG Y Y, JIANG H, ZHANG Y, et al. The dispersity-dependent interaction between montmorillonite supported nZVI and Cr(Ⅵ) in aqueous solution[J]. Chemical Engineering Journal, 2013, 229:412-419. [116] PANG H, WU Y, HUANG S, et al. Macroscopic and microscopic investigation of uranium elimination by Ca-Mg-Al-layered double hydroxide supported nanoscale zero valent iron[J]. Inorganic Chemistry Frontiers, 2018, 5:2657-2665. [117] LIU H, LI M, CHEN T, et al. New synthesis of nZVI/C composites as an efficient adsorbent for the uptake of U(Ⅵ) from aqueous solutions[J]. Environmental Science & Technology, 2017, 51:9227-9234. [118] ZHOU Y, GAO B, ZIMMERMAN A R, et al. Biochar-supported zerovalent iron for removal of various contaminants from aqueous solutions[J]. Bioresource Technology, 2014, 152:538-542. [119] DONG H, DENG J, XIE Y, et al. Stabilization of nanoscale zero-valent iron (nZVI) with modified biochar for Cr(Ⅵ) removal from aqueous solution[J]. Journal of Hazardous Materials, 2017, 332:79-86. [120] LI J, CHEN C, ZHANG R, et al. Nanoscale zero-valent iron particles supported on reduced graphene oxides by using a plasma technique and their application for removal of heavy-metal ions[J]. Chemistry-An Asian Journal, 2015, 10:1410-1417. [121] ZHENG Y, LIU J, LIANG J, et al. Graphitic carbon nitride materials:Controllable synthesis and applications in fuel cells and photocatalysis[J]. Energy & Environmental Science, 2012, 5:6717-6731. [122] TANG C, LING L,ZHANG W X. Pb(Ⅱ) deposition-reduction-growth onto iron nanoparticles induced by graphitic carbon nitride[J]. Chemical Engineering Journal, 2020, 387:124088. [123] BHOWMICK S, CHAKRABORTY S, MONDAL P, et al. Montmorillonite-supported nanoscale zero-valent iron for removal of arsenic from aqueous solution:Kinetics and mechanism[J]. Chemical Engineering Journal, 2014, 243:14-23. [124] LV X, XU J, JIANG G, et al. Removal of chromium(Ⅵ) from wastewater by nanoscale zero-valent iron particles supported on multiwalled carbon nanotubes[J]. Chemosphere, 2011, 85:1204-1209. [125] O'CARROLL D, SLEEP B, KROL M, et al. Nanoscale zero valent iron and bimetallic particles for contaminated site remediation[J]. Advances in Water Resources, 2013, 51:104-122. [126] LIU Y,LOWRY G V. Effect of particle age (Fe0 Content) and solution pH on nZVI reactivity:H2 evolution and TCE dechlorination[J]. Environmental Science & Technology, 2006, 40:6085-6090. [127] SHENG G, SHAO X, LI Y, et al. Enhanced removal of uranium(Ⅵ) by nanoscale zerovalent iron supported on Na-bentonite and an investigation of mechanism[J]. The Journal of Physical Chemistry A, 2014, 118:2952-2958. [128] 刘静, 刘爱荣, 张伟贤.纳米零价铁及其在环境介质中氧化后性质演变研究进展[J]. 环境化学, 2014, 33(4):576-583. LIU J, LIU A R, ZHANG W X. Review on transformation of oxidized nanoscale zero valent iron in environment media[J]. Environmental Chemistry, 2014, 33(4):576-583(in Chinese).
[129] LIU Z, ZHANG F, HOEKMAN S K, et al. Homogeneously dispersed zerovalent iron nanoparticles supported on hydrochar-derived porous carbon:simple, in situ synthesis and use for dechlorination of PCBs[J]. ACS Sustainable Chemistry & Engineering, 2016, 4:3261-3267. [130] LIU T, YANG X, WANG Z L, et al. Enhanced chitosan beads-supported Fe0-nanoparticles for removal of heavy metals from electroplating wastewater in permeable reactive barriers[J]. Water Research, 2013, 47:6691-6700. [131] SU Y, ZHANG Y, KE H, et al. Environmental remediation of chlorinated hydrocarbons using biopolymer stabilized iron loaded halloysite nanotubes[J]. ACS Sustainable Chemistry & Engineering, 2017, 5:10976-10985. [132] ZHAN J, ZHENG T, PIRINGER G, et al. Transport characteristics of nanoscale functional zerovalent iron/silica composites for in situ remediation of trichloroethylene[J]. Environmental Science & Technology, 2008, 42:8871-8876. [133] WEI A, MA J, CHEN J, et al. Enhanced nitrate removal and high selectivity towards dinitrogen for groundwater remediation using biochar-supported nano zero-valent iron[J]. Chemical Engineering Journal, 2018, 353:595-605. [134] JIA Z, SHU Y, HUANG R, et al. Enhanced reactivity of nZVI embedded into supermacroporous cryogels for highly efficient Cr(Ⅵ) and total Cr removal from aqueous solution[J]. Chemosphere, 2018, 199:232-242. [135] SHENG G, YANG P, TANG Y, et al. New insights into the primary roles of diatomite in the enhanced sequestration of UO22+ by zerovalent iron nanoparticles:An advanced approach utilizing XPS and EXAFS[J]. Applied Catalysis B-Environmental, 2016, 193:189-197. [136] LI Y, CHENG W, SHENG G, et al. Synergetic effect of a pillared bentonite support on Se(Ⅵ) removal by nanoscale zero valent iron[J]. Applied Catalysis B:Environmental, 2015, 174/175:329-335. [137] LIU Y, MAJETICH S A, TILTON R D, et al. TCE dechlorination rates, pathways, and efficiency of nanoscale iron particles with different properties[J]. Environmental Science & Technology, 2005, 39:1338-1345. [138] BAE S, COLLINS R N, WAITE T D, et al. Advances in surface passivation of nanoscale zerovalent iron:A critical review[J]. Environmental Science & Technology, 2018, 52:12010-12025. [139] RODRIGUES R, BETELU S, COLOMBANO S, et al. Reductive dechlorination of hexachlorobutadiene by a Pd/Fe microparticle suspension in dissolved lactic acid polymers:degradation mechanism and kinetics[J]. Industrial & Engineering Chemistry Research, 2017, 56:12092-12100. [140] DE TORO J A, VASILAKAKI M, LEE S S, et al. Remanence plots as a probe of spin disorder in magnetic nanoparticles[J]. Chemistry of Materials, 2017, 29:8258-8268. [141] LING X, LI J, ZHU W, et al. Synthesis of nanoscale zero-valent iron/ordered mesoporous carbon for adsorption and synergistic reduction of nitrobenzene[J]. Chemosphere, 2012, 87:655-660. [142] HOCH L B, MACK E J, HYDUTSKY B W, et al. Carbothermal synthesis of carbon-supported nanoscale zero-valent iron particles for the remediation of hexavalent chromium[J]. Environmental Science & Technology, 2008, 42:2600-2605. [143] HE F,ZHAO D. Hydrodechlorination of trichloroethene using stabilized Fe-Pd nanoparticles:Reaction mechanism and effects of stabilizers, catalysts and reaction conditions[J]. Applied Catalysis B:Environmental, 2008, 84:533-540. -
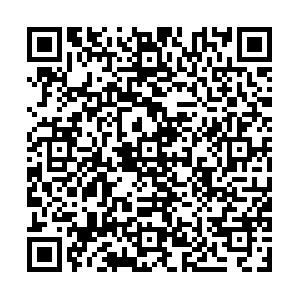
计量
- 文章访问数: 8586
- HTML全文浏览数: 8586
- PDF下载数: 384
- 施引文献: 0