转Cry1Ie基因玉米和转CP4-EPSPS基因大豆对斑马鱼的生态毒性
Ecotoxicological Effects of Transgenic Cry1Ie Maize and Transgenic CP4-EPSPS Soybean on Zebrafish
-
摘要: 为评估转Cry1Ie基因抗虫玉米和转CP4-EPSPS基因耐除草剂大豆对鱼类的生态毒理效应,以斑马鱼(Danio rerio)为受试动物,配制4种分别含有转基因玉米、转基因大豆以及相应非转基因亲本的试验饲料,并以商业饲料为对照,通过98 d喂养实验,调查斑马鱼的摄食、生长、繁殖和抗氧化酶活性,分析不同喂养阶段斑马鱼的组织病理和敏感蛋白mRNA表达水平。结果表明:抗虫转基因玉米和耐除草剂转基因大豆对斑马鱼的生长表现、肝脏、肠道和性腺的组织病理、产卵量和受精卵孵化率均无显著影响。转基因大豆组斑马鱼肝脏中的超氧化物歧化酶(SOD)活性显著高于非转基因大豆组和商业饲料组,且雄鱼显著高于雌鱼(P<0.05)。斑马鱼脏器组织中敏感蛋白mRNA的表达水平,没有随时间呈现明显的规律性变化,喂养时间与组别和性别之间存在显著的交互作用。试验饲料组斑马鱼的生长表现、肝脏中SOD活性以及mRNA表达量与商业饲料组相比有显著差异,可能与饲料的适口性和营养成分上的差异有关。总体上看,转Cry1Ie基因抗虫玉米和转CP4-EPSPS基因耐除草剂大豆对斑马鱼没有明显的生态毒性效应。Abstract: To evaluate the ecotoxicological effects of transgenic Cry1Ie insect-resistant maize and transgenic CP4-EPSPS herbicide-tolerant soybean on fish, zebrafish (Danio rerio) was used as a model animal and four experimental diets, including genetically modified (GM) maize, GM soybean, and corresponding near-isogenic non-GM lines were prepared respectively. A commercial feed was used as a reference diet. The feed intake, growth, reproduction, and antioxidant enzyme activity were investigated during a 98-day feeding trial. The histopathology and mRNA expression levels of sensitive protein were analyzed at different feeding stages. The results revealed that insect-resistant GM maize and herbicide-resistant GM soybean had no significant effects on growth performance, histopathology of the liver, intestinal tract, and brain, fecundity, and hatching rate of fertilized eggs. The activity of superoxide dismutase (SOD) was significantly higher in the liver of zebrafish fed with GM soybean compared with in those fed with the non-GM soybean and commercial diets, and was higher in male than in female fish (P<0.05). There were no obvious regular changes over time of mRNA expression levels of sensitive protein in zebrafish organ tissues. There were significant interaction effects between feeding duration, diet, and sex. When comparing experimental diets and commercial feed, we detected significant differences in growth performance, SOD activity in the liver, and mRNA expression levels, which may be related to the differences in palatability and nutrient composition of diets. In general, transgenic Cry1Ie insect-resistant maize and transgenic CP4-EPSPS herbicide-tolerant soybean have no obvious ecotoxicological effects on zebrafish.
-
Key words:
- insect-resistant GM maize /
- herbicide-resistant GM soybean /
- zebrafish /
- ecotoxicology
-
长江上游地区是我国重要的经济发展带,位于长江上游的中大型城市多以丘陵地貌为主,具有地势落差大、雨污收集速度快、地下水渗入率高等雨污排放特征[1-3]。城市污水处理厂是雨污治理的重要基础设施。一方面,污水处理厂减少了有机污染物、氮磷等污染物的排放,在水环境污染物控制中发挥重要作用[4-6]。城市污水处理厂累计削减的有机污染物 (以COD计) 占全国减排总量的70%以上,且占比逐年增加[7];另一方面,城市污水处理厂需消耗大量电能。污水处理厂的用电量约占全社会用电量的2.6‰[8],且占比逐年上升。此外,城市污水处理厂在深度脱氮除磷中,需使用大量的有机碳源和混凝剂[9-11]。
随着城市污水水量增加和污染物种类增多,污水处理厂面临严峻挑战。为保证出水水质达标,污水处理厂会考虑延长处理工艺单元链和增加了药剂的投加量,致使其运行成本增加[12-13]。本研究拟基于长江上游西南丘陵城市污水处理厂的运行优化需求,以泸州作为长江上游西南丘陵城市的代表,分析2020—2022年泸州中心区城市污水处理厂的电力、混凝剂和碳源使用量和使用效率,并与同期全国城市污水处理厂运行情况比较,以期掌握西南典型丘陵城市污水处理厂的用电量和药剂使用量特征,为污水处理厂运行优化提供参考。
1. 污水处理厂数据来源与运行特征
1.1 数据来源
本研究中泸州中心区5座城市污水处理厂的运行用电量、药剂投加量和进水与出水水质由泸州市住房和城乡建设局、泸州市兴泸水务 (集团) 股份有限公司提供,全国污水处理厂的运行数据来自住建部全国城镇污水处理管理信息系统和《中国城镇污水处理与再生利用发展报告 (1978-2020) 》。污水处理厂的用电量和药剂投加量为月累积用量;进水和出水水质指标为月均数据;统计期 (2020年1月—2022年4月) 内中位值用于定量讨论水质特征,5th~95th概率分布 (将数据按大小顺序排列的5%和95%分位值) 和箱式图用于表示水质数据分布特征。
1.2 泸州市城区污水处理厂基本情况
泸州市位于长江上游丘陵地区,其城市污水和初期雨水经由排水管网进入污水处理厂处理后再排入河流中。该市中心区共有5座城市污水处理厂,依次为纳溪污水处理厂、鸭儿凼污水处理厂、二道溪污水处理厂、城东污水处理厂和城南污水处理厂。5座污水处理厂的设计污水处理量、主体处理工艺和主要耗能单元如表1所示。
表 1 泸州中心区5座城市污水处理厂概况Table 1. Overview of the five urban wastewater treatment plants in the central area of Luzhou污水处理厂 设计污水处理量/(104 m3·d−1) 主体处理工艺和主要能耗单元 纳溪污水处理厂 2.75 曝气沉砂池-A2/O改良工艺-紫外消毒渠 鸭儿凼污水处理厂 8.0 曝气沉砂池-MBR-紫外消毒渠 二道溪污水处理厂 10.0 曝气沉砂池-A2/O-反硝化滤池-紫外消毒渠 城东污水处理厂 5.0 曝气沉砂池-A2/O改良工艺-D型滤池-紫外消毒渠 城南污水处理厂 5.0 曝气沉砂池-A2/O改良工艺-D型滤池-紫外消毒渠 注:A2/O改良工艺即在厌氧池前端增加了预反硝化单元。 1.3 污水处理厂处理水量与进出水水质
污水处理厂的处理水量和运行负荷如图1所示。一方面,污水处理厂水量突然升高情况较多,与该地区的降雨频繁密切关联;另一方面,水量随季节性波动明显,二道溪、城东和城南污水处理厂在7—9月份的水量较大[14]。从2021年开始,城南污水处理厂和城东污水处理厂处理水量长期大于设计处理量,主体工艺处于高负荷运行状态。此外,表2展示了5座污水处理厂进出水水质,出水符合《城镇污水处理厂污染物排放标准》 (GB 18918-2002) 一级A排放标准,但距离地表Ⅲ类水相关指标限值尚有一定差距。
表 2 泸州中心区城市污水处理厂进水和出水水质特征 (2020年1月—2022年4月)Table 2. Quality characteristics of inlet and outlet water of urban wastewater treatment plants in the central area of Luzhou (2020-01—2022-04)污水 处理厂 BOD5/(mg·L−1) TN/(mg·L−1) [NH3-N]/(mg·L−1) TP/(mg·L−1) 进水 出水 进水 出水 进水 出水 进水 出水 纳溪污水处理厂 198.47±60.79 12.03±3.87 27.80±6.67 9.57±1.10 18.70±5.72 0.61±0.45 2.79±0.72 0.21±0.06 鸭儿凼污水处理厂 197.01±55.66 11.77±1.79 29.85±5.18 7.83±1.54 23.21±2.95 0.51±0.32 3.40±1.01 0.24±0.06 二道溪污水处理厂 231.52±55.01 11.26±2.61 29.85±5.18 7.83±1.54 28.39±9.40 0.04±0.39 3.54±0.85 0.25±0.09 城东污水处理厂 182.33±78.28 19.86±3.55 33.18±8.68 10.20±1.21 25.69±6.69 0.04±0.28 3.28±1.08 0.31±0.03 城南污水处理厂 216.65±65.50 11.97±2.99 28.97±7.55 9.25±1.49 20.73±6.04 0.03±0.03 2.50±0.77 0.24±0.07 2. 结果与讨论
2.1 污水处理厂运行用电量与用电效率
1) 运行用电量。用电量是污水处理厂的主要运行成本之一,亟需识别西南典型丘陵城市污水处理厂的用电特征和用电效率[15-16]。总用电量 (Q,104 kW·h) 、单位处理水量的用电量 (QV,kW·h·m−3) 和单位污染物去除量的用电量 (QCOD和QNH3-N,分别指去除污水中单位质量有机污染物 (以COD计) 或NH3-N的用电量,kW·h·kg−1) ,常用于表示用电特征和用电效率,计算方法如式 (1)~(3) 。
Qv(kW⋅h⋅m−3)=总用电量(kW⋅h)处理水量(m3) (1) Qc(kW⋅h⋅kg−3)=总用电量(kW⋅h)COD削减量(kg) (2) QNH3−N(kW⋅h⋅kg−3)=总用电量(kW⋅h)NH3−N削减量(kg) (3) 泸州中心区5座城市污水处理厂的总用电量Q为20.0~110.9 104 kW·h (5th~95th) ,如图2 (a) 所示。鸭儿凼污水处理厂的Q值最大 (中位值105.75 104 kW·h) ,其次为二道溪污水处理厂 (中位值 85.10 104 kW·h) 。城东和城南污水处理厂的处理水量和主体处理工艺相同,但城南污水处理厂Q的中位值为51.01 104 kW·h ,高于城东污水处理厂 (中位值47.61 104 kW·h) 。在污水处理厂的实际运行当中,曝气是主要的耗能工艺。此外,5座城市污水处理厂均在三级处理流程中设置了紫外消毒渠处理单元,这也会增加污水处理厂的总用电量。伴随着污水处理工艺链的延长,电耗的总量和来源也逐渐增加。
泸州中心区5座城市污水处理厂的QV为0.25~0.52 kW·h·m−3 (5th~95th) ,如图2(b)所示。鸭儿凼的QV值最高 (中位值0.46 kW·h·m−3) ,与其膜生物反应器 (membrane bioreactor,MBR) 处理工艺能耗较高有关[17]。城东污水处理厂的QV值最低 (中位值0.28 kW·h·m−3) ,且显著低于城南污水处理厂 (中位值 0.36 kW·h·m−3) 。城东污水处理厂与城南污水处理厂的处理水量相近、工艺相同,QV差异表明进水水质、运营操作等因素会导致用电效率的差异。
泸州中心区城市污水处理厂的QCOD和QNH3-N分别如图2(c)和图2(d)所示。QCOD为1.12~3.55 kW·h·kg−1 (5th~95th) 。其中,鸭儿凼污水处理厂的QCOD最高 (中位值2.58 kW·h·kg−1) ,城东污水处理厂QCOD稍高于城南污水处理厂且分布范围较大 (中位值1.94 kW·h·kg−1) 。QNH3-N为9.64~30.6 kW·h·kg−1 (5th~95th) 。鸭儿凼污水处理厂的QNH3-N最大 (中位值20.57 kW·h·kg−1) ,而城东污水处理厂的QNH3-N最小 (中位值11.23 kW·h·kg−1) ,且显著低于城南污水处理厂 (中位值 17.39 kW·h·kg−1)。
2) 运行用电效率。分析2020—2021年全国5 799座城镇污水处理厂处理用电量 (图3(a)) 。QV为0.16~1.20 kW·h·m−3 (5th~95th) ,中位值和平均值分别为0.36和0.47 kW·h·m−3。80%的污水处理厂的QV低于0.60 kW·h·m−3。此外,泸州中心区5座城市污水处理厂的QV均优于全国平均值,但差于前28%的污水处理厂。其中,鸭儿凼、二道溪和城南污水处理厂的QV差于国内50%以上的污水处理厂。由此说明,泸州中心城区城市污水处理厂的用电效率整体较高,但仍可通过处理工艺优化和升级进一步提高用电效率[18-19]。
泸州是典型的西南丘陵城市。进一步比较其与西南地区和其他地区的用电效率,结果如图3(b)所示。华北地区的QV值最高 (中位值 0.61 kW·h·m−3) ,西南地区、西北地区和华东地区的QV值为中等 (中位值 0.36~0.51 kW·h·m−3) 。东北地区、华中地区和华南地区的QV值最低 (中位值 0.27~0.36 kW·h·m−3) 。与各地区典型运行效率相比,泸州市中心城区5座污水处理厂的QV优于华北地区、西北地区和西南地区的QV。各地区Qv的存在较显著的差异,与污水进水水质、处理工艺和气候紧密关联[20-21]。
为增加运行用电效率,污水处理厂从厂区设计方面应该结合所处的地形特点,在处理单元高程布置中采用合理的布局,以减少提升泵的使用。此外,鼓风机和搅拌器是主要耗能设备,从选购的角度来说,应考虑实际处理水量和水质,选择型号适宜的设备;从布置的角度来说,精准曝气以增加溶解氧的传质速率可提升运行用电效率[22]。
2.2 污水处理厂除磷混凝剂用量与使用效率
1) 除磷混凝剂用量。泸州市中心城区污水处理厂投加的混凝药剂为聚合硫酸铁 (polyferric sulfate,PFS) 。2020—2022年5座城市污水处理厂的单位处理水量的PFS投加量 (PFSV,mg·L−1) 和单位总磷 (TP) 去除量的PFS投加量 (PFSTP,mg·mg−1) 如图4所示。鸭儿凼和二道溪污水处理厂的PFSV较高,为48.42~53.57 mg·L−1;城东和城南污水处理厂的PFSV较低,为25.11~26.98 mg·L−1。
图 4 泸州中心区城市污水处理厂单位处理水量的PFS投加量 (PFSV) 和单位总磷去除量的PFS投加量 (PFSTP) 时间变化和统计分布 (2020年1月—2022年4月)Figure 4. Temporal variation and statistical distribution of PFS dosage per unit treated water volume (PFSV) and PFS dosage per unit total phosphorus removal (PFSTP) of urban wastewater treatment plants in the central area of Luzhou (2020-01—2022-04)PFSTP与进水和出水水质密切相关。泸州中心城区污水处理厂出水TP均满足一级A排放要求。进一步分析发现,泸州中心城区5座污水处理厂的PFSTP为2.67~30.41 mg·mg−1 (5th~95th) 。城东污水处理厂PFS投加质量分数较低,但同时PFSTP仅为9.41 mg·mg−1;鸭儿凼不仅PFS投加质量分数较高,同时PFSTP达到19.16 mg·mg−1。这与鸭儿凼污水处理厂二级出水的磷形态 (偏磷酸盐或溶解态为主) 等有关,需进一步开展水质分析来提高除磷工艺优化[23-24]。值得注意的是,城东污水处理厂和城南污水处理厂的工艺和设计规模相同,但城南污水处理厂的PFSTP显著较高,为13.34 mg·mg−1。这可能是由于工艺操作和水中磷形态不同。如PFS对偏磷酸盐的去除效果较差,对磷形态分析和操作条件优化将有助于提高除磷效率和混凝剂投加效率[25]。
2) 除磷混凝剂使用效率。比较泸州市中心城区5座污水处理厂与国内其他污水处理厂的混凝剂用量,结果如表3所示。北京市吴家村污水处理厂和上海市城投污水处理厂投加的混凝剂为PFS,但其PFSTP是泸州市中心城区污水处理厂的1.36和2.06倍;昆明市第三污水处理厂投加的混凝剂为聚合氯化铝 (polyaluminium chloride,PAC) ,单位TP去除量的PAC投加量 (PACTP) 为11.1 mg·mg−1。混凝剂使用效率比较结果表明,泸州市中心城区的PFS使用效率较高;由于PFS与PAC的性质差异,混凝剂的处理效率和使用效率存在差异。后续可基于混凝剂的单位除磷效率,进行混凝剂种类、操作条件和投加量等工艺条件优化[26]。污水处理厂的PFSTP与进出水TP关系如表3和图5所示,拟合结果表明其线性相关性较差。这说明污水处理厂的在三级处理化学除磷过程中,混凝剂使用效率PFSTP与进出水TP线性不相关[27]。
表 3 典型城市污水厂处理厂混凝剂药耗水平Table 3. Coagulant consumption level in a typical urban wastewater treatment plants2.3 污水处理厂运行碳源投加量
1) 碳源投加量。为实现高效除氮,污水处理厂通常需要补充外部碳源[30-31]。如图6 (a) 所示,泸州市中心区5座城市污水处理厂单位处理水量的碳源投加量 (CV,折合COD计) 为1.45~161.38 g·m−3 (5th~95th) 。污水处理厂之间CV差别较大,其中纳溪污水处理厂投加的碳源较少 (中位值14.04 g·m−3) ,城东污水处理厂投加的碳源较多 (中位值 96.43 g·m−3) 。污水处理厂的CV差异与进水碳源、形态、污水处理工艺等因素相关。在泸州市的中心区污水处理厂中,单位总氮 (TN) 去除量对应碳源投加量 (CTN,以COD计) 为0.13~6.55 g·g−1 (5th~95th) (图6 (b) )。污水处理厂间的CTN差异规律与CV相似。城东污水处理厂的CTN较高 (中位值为4.88 g·g−1) ,二道溪污水处理厂较低 (中位值为0.60 g·g−1) 。
2) 碳源使用效率。2020年全国2 347座城镇污水处理厂的CV和CTN如图7所示,CV为1.4~154.2 g·m−3 (5th~95th) ,中位值为20.2 g·m−3 (图7(a)) ; CTN为0.04~4.67 g·g−1 (5th~95th) ,中间值为4.84 g·g−1 (图7(b)) 。泸州中心区的5座污水处理厂CV和CTN均差于国内50%以上的污水处理厂,这说明泸州市这5座污水处理厂的碳源使用效率仍有较大提升空间。
为探究碳源使用效率CTN与进出水TN的相关性,对CTN与进出水TN进行线性拟合,结果如图8所示,这说明污水处理厂在外加碳源增强反硝化脱氮过程中,碳源使用效率CTN与进出水TN线性不相关。为提升碳源的使用效率或减少碳源的使用量,可考虑从运行优化、工艺升级与协同两个方面入手。以往污水处理厂投加外加碳源是凭借经验控制投加量,这会导致在维持出水TN达标的情况下,部分碳源可能浪费且增加水体BOD5。而精准加碳可提升碳源的使用效率,可通过对硝酸盐和亚硝酸盐质量浓度的在线监测和反馈调节来实现精准加碳。此外,工艺升级与协同可减少碳源的使用量,采用短程硝化工艺使得氮元素以亚硝酸根的形式存在,进而减少还原过程中的碳源投加量。此外,反硝化滤池工艺的增加和协同不仅可减少碳源在A2/O工艺的投加量,还可维持出水TN稳定性。
3. 结论
1) 西南丘陵城市泸州市中心区城市污水处理厂的电力、混凝剂和碳源的用量具有显著季节特征,夏季降雨较多时的用电量和药剂用量较高。2) 在用电量方面,泸州市中心城区污水处理厂的QV为0.25~0.52 kW·h·m−3,用电效率较好,位于全国28%~66%。其中,鸭儿凼污水处理厂的QV较高 (中位值 0.46 kW·h·m−3) ,与其膜生物处理工艺有关。城东和城南污水处理厂的设计处理水量和工艺相同,但QV和QNH3~N差异较大,主要原因为进水中污染物的种类和[NH3-N]差异较大。因此,污水处理厂用电量、用电效率与水量相关,也与进水出水水质、操作条件等相关。3) 在药剂投加量方面,泸州市中心城区污水处理厂以PFS为除磷混凝剂,PFSTP效率较高,为2.67~30.41 mg·mg−1;泸州市中心区城市污水处理厂的碳源使用率较低,位于全国44%~95%,需通过对进水中硝酸盐与亚硝酸盐质量浓度进行监测、升级脱氮工艺和外加碳源精准投加等方式来提升碳源使用效率。
-
国际农业生物技术应用服务组织. 2019年全球生物技术/转基因作物商业化发展态势[J]. 中国生物工程杂志, 2021, 41(1):114-119 International Service for the Acquisition of Agri-Biotech Applications. The global status of commercialized biotech/GM crops in 2019[J]. China Biotechnology, 2021, 41(1):114-119(in Chinese)
Strain K E, Whiting S A, Lydy M J. Laboratory and field validation of a Cry1Ab protein quantitation method for water[J]. Talanta, 2014, 128:109-116 Whiting S A, Strain K E, Campbell L A, et al. A multi-year field study to evaluate the environmental fate and agronomic effects of insecticide mixtures[J]. Science of the Total Environment, 2014, 497-498:534-542 Pott A, Otto M, Schulz R. Impact of genetically modified organisms on aquatic environments:Review of available data for the risk assessment[J]. Science of the Total Environment, 2018, 635:687-698 Douville M, Gagné F, André C, et al. Occurrence of the transgenic corn cry1Ab gene in freshwater mussels (Elliptio complanata) near corn fields:Evidence of exposure by bacterial ingestion[J]. Ecotoxicology and Environmental Safety, 2009, 72(1):17-25 陈秀萍, 王加美, 朱昊俊, 等. 转Bt基因作物对水生生态系统的影响研究进展[J]. 应用与环境生物学报, 2013, 19(4):569-574 Chen X P, Wang J M, Zhu H J, et al. Progress in effects of transgenic Bt crops on the aquatic ecosystem[J]. Chinese Journal of Applied and Environmental Biology, 2013, 19(4):569-574(in Chinese)
Harris J H. The use of fish in ecological assessments[J]. Austral Ecology, 1995, 20(1):65-80 董姗姗, 章嫡妮, 张振华, 等. 转基因作物对鱼类的生态毒理效应研究综述[J]. 生态毒理学报, 2017, 12(5):26-34 Dong S S, Zhang D N, Zhang Z H, et al. Overview of ecotoxicological effects of genetically modified crops on fish[J]. Asian Journal of Ecotoxicology, 2017, 12(5):26-34(in Chinese)
Sissener N H, Sanden M, Krogdahl Å, et al. Genetically modified plants as fish feed ingredients[J]. Canadian Journal of Fisheries and Aquatic Sciences, 2011, 68(3):563-574 Hemre G I, Sagstad A, Bakke-Mckellep A M, et al. Nutritional, physiological, and histological responses in Atlantic salmon, Salmo salar L. fed diets with genetically modified maize[J]. Aquaculture Nutrition, 2007, 13(3):186-199 Gu J N, Bakke A M, Valen E C, et al. Bt-maize (MON810) and non-GM soybean meal in diets for Atlantic salmon (Salmo salar L.) juveniles-Impact on survival, growth performance, development, digestive function, and transcriptional expression of intestinal immune and stress responses[J]. PLoS One, 2014, 9(6):e99932 Schlegel A, Stainier D Y R. Lessons from "lower" organisms:What worms, flies, and zebrafish can teach us about human energy metabolism[J]. PLoS Genetics, 2007, 3(11):e199 Seth A, Stemple D L, Barroso I. The emerging use of zebrafish to model metabolic disease[J]. Disease Models & Mechanisms, 2013, 6(5):1080-1088 Gabriëls I, Vergauwen L, de Boevre M, et al. Optimizing the use of zebrafish feeding trials for the safety evaluation of genetically modified crops[J]. International Journal of Molecular Sciences, 2019, 20(6):1472 Rayan A M, Nigussie F, Abbott L C. Safety evaluation of stacked genetically modified corn event (MON89034×MON88017) using zebrafish as an animal model[J]. Food and Nutrition Sciences, 2015, 6(14):1285-1295 National Research Council. Nutrient requirement of fish[R]. Washington DC:National Academy Press, 1993 董姗姗, 章嫡妮, 张振华, 等. 转mCry1Ac基因玉米BT799对斑马鱼的生态毒理学效应[J]. 应用生态学报, 2019, 30(8):2845-2853 Dong S S, Zhang D N, Zhang Z H, et al. Ecotoxicological effects of transgenic mCry1Ac maize (BT799) on zebrafish[J]. Chinese Journal of Applied Ecology, 2019, 30(8):2845-2853(in Chinese)
中华人民共和国国家质量监督检验检疫总局, 中国国家标准化管理委员会. 饲料卫生标准:GB 13078-2017[S]. 北京:中华人民共和国国家质量监督检验检疫总局, 中国国家标准化管理委员会, 2017 General Administration of Quality Supervision, Inspection and Quarantine of the People's Republic of China, Standardization Administration of China. Hygienical standard for feeds:GB 13078-2017[S]. Beijing:General Administration of Quality Supervision, Inspection and Quarantine of the People's Republic of China, Standardization Administration of China, 2017 (in Chinese)
Sanden M, Krogdahl A, Bakke-Mckellep A M, et al. Growth performance and organ development in Atlantic salmon, Salmo salar L. parr fed genetically modified (GM) soybean and maize[J]. Aquaculture Nutrition, 2006, 12(1):1-14 Sissener N H, Johannessen L E, Hevrøy E M, et al. Zebrafish (Danio rerio) as a model for investigating the safety of GM feed ingredients (soya and maize); performance, stress response and uptake of dietary DNA sequences[J]. The British Journal of Nutrition, 2010, 103(1):3-15 Nordgarden U, Oppedal F, Taranger G L, et al. Seasonally changing metabolism in Atlantic salmon (Salmo salar L.) I-Growth and feed conversion ratio[J]. Aquaculture Nutrition, 2003, 9(5):287-293 Sissener N H, Sanden M, Bakke A M, et al. A long term trial with Atlantic salmon (Salmo salar L.) fed genetically modified soy; focusing general health and performance before, during and after the parr-smolt transformation[J]. Aquaculture, 2009, 294(1-2):108-117 Sagstad A, Sanden M, Krogdahl, et al. Organs development, gene expression and health of Atlantic salmon (Salmo salar L.) fed genetically modified soybeans compared to the near-isogenic non-modified parental line[J]. Aquaculture Nutrition, 2008, 14(6):556-572 Sissener N H, Bakke A M, Gu J, et al. An assessment of organ and intestinal histomorphology and cellular stress response in Atlantic salmon (Salmo salar L.) fed genetically modified Roundup Ready® soy[J]. Aquaculture, 2009, 298(1-2):101-110 Sanden M, Ornsrud R, Sissener N H, et al. Cross-generational feeding of Bt (Bacillus thuringiensis)-maize to zebrafish (Danio rerio) showed no adverse effects on the parental or offspring generations[J]. The British Journal of Nutrition, 2013, 110(12):2222-2233 Goolish E M, Okutake K, Lesure S. Growth and survivorship of larval zebrafish Danio rerio on processed diets[J]. North American Journal of Aquaculture, 1999, 61(3):189-198 Sanden M, Jørgensen S, Hemre G I, et al. Zebrafish (Danio rerio) as a model for investigating dietary toxic effects of deoxynivalenol contamination in aquaculture feeds[J]. Food and Chemical Toxicology, 2012, 50(12):4441-4448 Iwashita Y, Yamamoto T, Furuita H, et al. Influence of certain soybean antinutritional factors supplemented to a casein-based semipurified diet on intestinal and liver morphology in fingerling rainbow trout Oncorhynchus mykiss[J]. Fisheries Science, 2008, 74(5):1075-1082 Nordrum S, Bakke-Mckellep A M, Krogdahl Å, et al. Effects of soybean meal and salinity on intestinal transport of nutrients in Atlantic salmon (Salmo salar L.) and rainbow trout (Oncorhynchus mykiss)[J]. Comparative Biochemistry and Physiology Part B:Biochemistry and Molecular Biology, 2000, 125(3):317-335 Duke S O, Rimando A M, Pace P F, et al. Isoflavone, glyphosate, and aminomethylphosphonic acid levels in seeds of glyphosate-treated, glyphosate-resistant soybean[J]. Journal of Agricultural and Food Chemistry, 2003, 51(1):340-344 王德鑫. 黄豆苷元对尼罗罗非鱼(Oreochromis niloticus)生长的影响及其机制研究[D]. 青岛:中国海洋大学, 2014:5-7 Wang D X. The research of effects and mechanisms of dietary daidzein on the growth of Nile tilapia (Oreochromis niloticus)[D]. Qingdao:Ocean University of China, 2014:5 -7(in Chinese)
Bennetau-Pelissero C, Breton B B, Bennetau B, et al. Effect of genistein-enriched diets on the endocrine process of gametogenesis and on reproduction efficiency of the rainbow trout Oncorhynchus mykiss[J]. General and Comparative Endocrinology, 2001, 121(2):173-187 Pietsch C, Katzenback B A, Garcia-Garcia E, et al. Acute and subchronic effects on immune responses of carp (Cyprinus carpio L.) after exposure to deoxynivalenol (DON) in feed[J]. Mycotoxin Research, 2015, 31(3):151-164 Ryerse I A, Hooft J M, Bureau D P, et al. Diets containing corn naturally contaminated with deoxynivalenol reduces the susceptibility of rainbow trout (Oncorhynchus mykiss) to experimental Flavobacterium psychrophiluminfection[J]. Aquaculture Research, 2016, 47(3):787-796 Anater A, Manyes L, Meca G, et al. Mycotoxins and their consequences in aquaculture:A review[J]. Aquaculture, 2016, 451:1-10 -
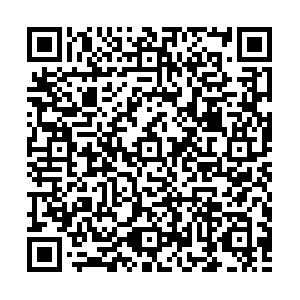
计量
- 文章访问数: 2294
- HTML全文浏览数: 2294
- PDF下载数: 36
- 施引文献: 0